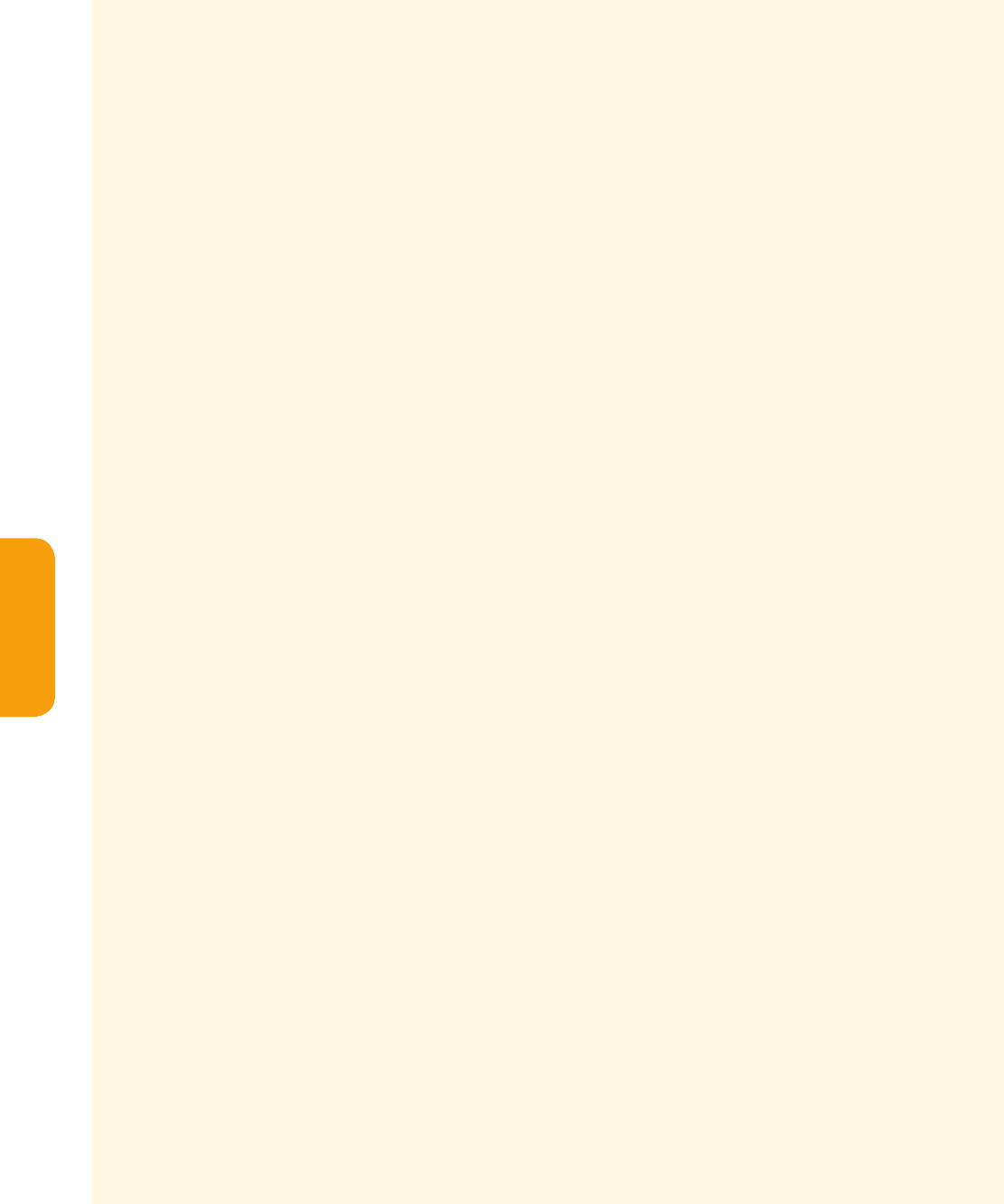
Frequently Asked Questions
FAQ
34
Frequently Asked Questions
FAQ 5.4 | What Are Carbon Budgets?
There are several types of carbon budgets. Most often, the term refers to the total net amount of carbon dioxide
(CO
2
) that can still be emitted by human activities while limiting global warming to aspecified level (e.g.,1.5°C
or 2°C above pre-industrial levels). This is referred to as the ‘remaining carbon budget’. Several choices and value
judgements have to be made before it can be unambiguously estimated. When the remaining carbon budget is
combined with all past CO
2
emissions to date, a‘total carbon budget’ compatible with aspecific global warming
limit can also be defined. Athird type of carbon budget is the ‘historical carbon budget’, which is ascientific way
to describe all past and present sources and sinks of CO
2
.
The term remaining carbon budget is used to describe the total net amount of CO
2
that human activities can
still release into the atmosphere while keeping global warming to aspecified level, like 1.5°C or 2°C relative
to pre-industrial temperatures. Emissions of CO
2
from human activities are the main cause of global warming.
Aremaining carbon budget can be defined because of the specific way CO
2
behaves in the Earth system. That
is, global warming is roughly linearly proportional to the total net amount of CO
2
emissions that are released
into the atmosphere by human activities –also referred to as cumulative anthropogenic CO
2
emissions. Other
greenhouse gases behave differently and have to be accounted for separately.
The concept of a remaining carbon budget implies that, to stabilize global warming at any particular level,
global emissions of CO
2
need to be reduced to net zero levels at some point. ‘Net zero CO
2
emissions’ describes
a situation where all the anthropogenic emissions of CO
2
are counterbalanced by deliberate anthropogenic
removals so that, on average, no CO
2
is added or removed from the atmosphere by human activities. Atmospheric
CO
2
concentrations in such asituation would gradually decline to along-term stable level as excess CO
2
in the
atmosphere is taken up by ocean and land sinks (see FAQ 5.1). The concept of aremaining carbon budget also
means that, if CO
2
emissions reductions are delayed, deeper and faster reductions are needed later to stay within
the same budget. If the remaining carbon budget is exceeded, this will result in either higher global warming
or aneed to actively remove CO
2
from the atmosphere to reduce global temperatures back down to the desired
level (see FAQ 5.3).
Estimating the size of remaining carbon budgets depends on aset of choices. These choices include: (1)theglobal
warming level that is chosen as alimit (for example, 1.5°C or 2°C relative to pre-industrial levels); (2) the probability
with which we want to ensure that warming is held below that limit (for example, aone-in-two, two-in-three,
or higher chance), and (3) how successful we are in limiting emissions of other greenhouse gases that affect the
climate, such as methane or nitrous oxide. These choices can be informed by science, but ultimately represent
subjective choices. Once these choices have been made, to estimate the remaining carbon budget for agiven
temperature goal, we can combine knowledge about: how much our planet has warmed already; the amount
of warming per cumulative tonne of CO
2
; and the amount of warming that is still expected once global net
CO
2
emissions are brought down to zero. For example, to limit global warming to 1.5°C above pre-industrial
levels with either a one-in-two (50%) or two-in-three (67%) chance, the remaining carbon budgets amount
to 500and 400 billion tonnes of CO
2,
respectively, from 1January 2020 onward (FAQ 5.4, Figure1). Currently,
human activities are emitting around 40 billion tonnes of CO
2
into the atmosphere in asingle year.
The remaining carbon budget depends on how much the world has already warmed to date. This past warming
is caused by historical emissions, which are estimated by looking at the historical carbon budget –a scientific way
to describe all past and present sources and sinks of CO
2
. It describes how the CO
2
emissions from human activities
have redistributed across the various CO
2
reservoirs of the Earth system. These reservoirs are the ocean, the land
vegetation, and the atmosphere (into which CO
2
was emitted). The share of CO
2
that is not taken up by the
ocean or the land, and that thus increases the concentration of CO
2
in the atmosphere, causes global warming.
The historical carbon budget tells us that, of the about 2560 billion tonnes of CO
2
that were released into the
atmosphere by human activities between the years 1750 and 2019, about aquarter were absorbed by the ocean
(causing ocean acidification) and about athird by the land vegetation. About 45% of these emissions remain in
the atmosphere (see FAQ 5.1). Adding these historical CO
2
emissions to estimates of remaining carbon budgets
allows an estimate of the total carbon budget consistent with aspecific global warming level.