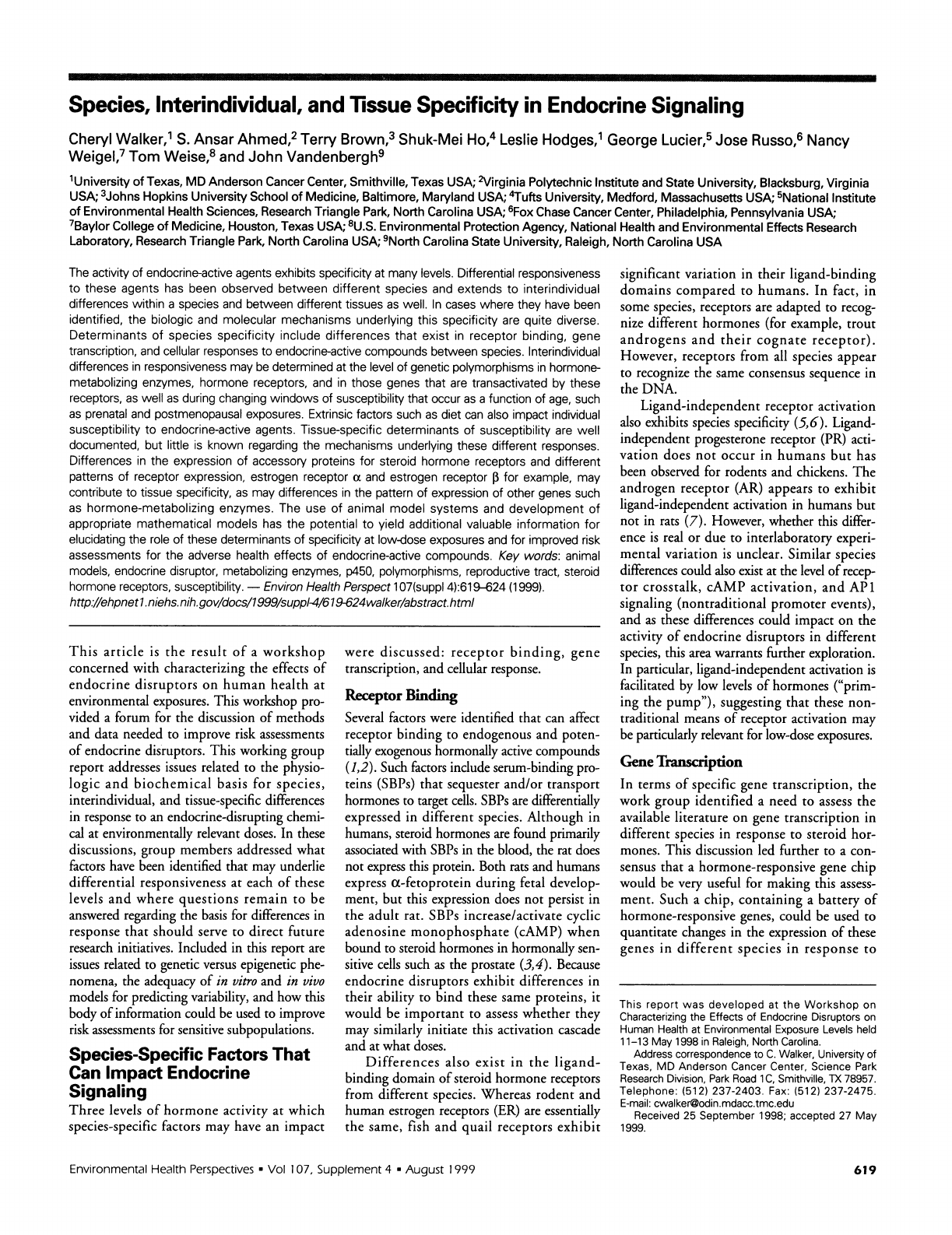
Species,
Interindividual,
and
Tissue
Specificity
in
Endocrine
Signaling
Cheryl
Walker,1
S.
Ansar
Ahmed,2
Terry
Brown,3
Shuk-Mei
Ho,4
Leslie
Hodges,1
George
Lucier,5
Jose
Russo,6
Nancy
Weigel,7
Tom
Weise,8
and
John
Vandenbergh9
'University
of
Texas,
MD
Anderson
Cancer
Center,
Smithville,
Texas
USA;
2Virginia
Polytechnic
Institute
and
State
University,
Blacksburg,
Virginia
USA;
3Johns
Hopkins
University
School
of
Medicine,
Baltimore,
Maryland
USA;
4Tufts
University,
Medford,
Massachusetts
USA;
5National
Institute
of
Environmental
Health
Sciences,
Research
Triangle
Park,
North
Carolina
USA;
6Fox
Chase
Cancer
Center,
Philadelphia,
Pennsylvania
USA;
7Baylor
College
of
Medicine,
Houston,
Texas
USA;
8U.S.
Environmental
Protection
Agency,
National
Health
and
Environmental
Effects
Research
Laboratory,
Research
Triangle
Park,
North
Carolina
USA;
9North
Carolina
State
University,
Raleigh,
North
Carolina
USA
The
activity
of
endocrine-active
agents
exhibits
specificity
at
many
levels.
Differential
responsiveness
to
these
agents
has
been
observed
between
different
species
and
extends
to
interindividual
differences
within
a
species
and
between
different
tissues
as
well.
In
cases
where
they
have
been
identified,
the
biologic
and
molecular
mechanisms
underlying
this
specificity
are
quite
diverse.
Determinants
of
species
specificity
include
differences
that
exist
in
receptor
binding,
gene
transcription,
and
cellular
responses
to
endocrine-active
compounds
between
species.
Interindividual
differences
in
responsiveness
may
be
determined
at
the
level
of
genetic
polymorphisms
in
hormone-
metabolizing
enzymes,
hormone
receptors,
and
in
those
genes
that
are
transactivated
by
these
receptors,
as
well
as
during
changing
windows
of
susceptibility
that
occur
as
a
function
of
age,
such
as
prenatal
and
postmenopausal
exposures.
Extrinsic
factors
such
as
diet
can
also
impact
individual
susceptibility
to
endocrine-active
agents.
Tissue-specific
determinants
of
susceptibility
are
well
documented,
but
little
is
known
regarding
the
mechanisms
underlying
these
different
responses.
Differences
in
the
expression
of
accessory
proteins
for
steroid
hormone
receptors
and
different
patterns
of
receptor
expression,
estrogen
receptor
a
and
estrogen
receptor
,B
for
example,
may
contribute
to
tissue
specificity,
as
may
differences
in
the
pattern
of
expression
of
other
genes
such
as
hormone-metabolizing
enzymes.
The
use
of
animal
model
systems
and
development
of
appropriate
mathematical
models
has
the
potential
to
yield
additional
valuable
information
for
elucidating
the
role
of
these
determinants
of
specificity
at
low-dose
exposures
and
for
improved
risk
assessments
for
the
adverse
health
effects
of
endocrine-active
compounds.
Key
words:
animal
models,
endocrine
disruptor,
metabolizing
enzymes,
p450,
polymorphisms,
reproductive
tract,
steroid
hormone
receptors,
susceptibility.
-
Environ
Health
Perspect
1
07(suppl
4):619-624
(1999).
http.//ehpnet1.
niehs.
nih.gov/docs/1999/suppl-4/619624walker/abstract.html
This
article
is
the
result
of
a
workshop
concerned
with
characterizing
the
effects
of
endocrine
disruptors
on
human
health
at
environmental
exposures.
This
workshop
pro-
vided
a
forum
for
the
discussion
of
methods
and
data
needed
to
improve
risk
assessments
of
endocrine
disruptors.
This
working
group
report
addresses
issues
related
to
the
physio-
logic
and
biochemical
basis
for
species,
interindividual,
and
tissue-specific
differences
in
response
to
an
endocrine-disrupting
chemi-
cal
at
environmentally
relevant
doses.
In
these
discussions,
group
members
addressed
what
factors
have
been
identified
that
may
underlie
differential
responsiveness
at
each
of
these
levels
and
where
questions
remain
to
be
answered
regarding
the
basis
for
differences
in
response
that
should
serve
to
direct
future
research
initiatives.
Included
in
this
report
are
issues
related
to
genetic
versus
epigenetic
phe-
nomena,
the
adequacy
of
in
vitro
and
in
vivo
models
for
predicting
variability,
and
how
this
body
of
information
could
be
used
to
improve
risk
assessments
for
sensitive
subpopulations.
Species-Specific
Factors
That
Can
Impact
Endocrine
Signaling
Three
levels
of
hormone
activity
at
which
species-specific
factors
may
have
an
impact
were
discussed:
receptor
binding,
gene
transcription,
and
cellular
response.
Recptor
Binding
Several
factors
were
identified
that
can
affect
receptor
binding
to
endogenous
and
poten-
tially
exogenous
hormonally
active
compounds
(1,2).
Such
factors
include
serum-binding
pro-
teins
(SBPs)
that
sequester
and/or
transport
hormones
to
target
cells.
SBPs
are
differentially
expressed
in
different
species.
Although
in
humans,
steroid
hormones
are
found
primarily
associated
with
SBPs
in
the
blood,
the
rat
does
not
express
this
protein.
Both
rats
and
humans
express
a-fetoprotein
during
fetal
develop-
ment,
but
this
expression
does
not
persist
in
the
adult
rat.
SBPs
increase/activate
cyclic
adenosine
monophosphate
(cAMP)
when
bound
to
steroid
hormones
in
hormonally
sen-
sitive
cells
such
as
the
prostate
(3,4).
Because
endocrine
disruptors
exhibit
differences
in
their
ability
to
bind
these
same
proteins,
it
would
be
important
to
assess
whether
they
may
similarly
initiate
this
activation
cascade
and
at
what
doses.
Differences
also
exist
in
the
ligand-
binding
domain
of
steroid
hormone
receptors
from
different
species.
Whereas
rodent
and
human
estrogen
receptors
(ER)
are
essentially
the
same,
fish
and
quail
receptors
exhibit
significant
variation
in
their
ligand-binding
domains
compared
to
humans.
In
fact,
in
some
species,
receptors
are
adapted
to
recog-
nize
different
hormones
(for
example,
trout
androgens
and
their
cognate
receptor).
However,
receptors
from
all
species
appear
to
recognize
the
same
consensus
sequence
in
the
DNA.
Ligand-independent
receptor
activation
also
exhibits
species
specificity
(5,6).
Ligand-
independent
progesterone
receptor
(PR)
acti-
vation
does
not
occur
in
humans
but
has
been
observed
for
rodents
and
chickens.
The
androgen
receptor
(AR)
appears
to
exhibit
ligand-independent
activation
in
humans
but
not
in
rats
(7).
However,
whether
this
differ-
ence
is
real
or
due
to
interlaboratory
experi-
mental
variation
is
unclear.
Similar
species
differences
could
also
exist
at
the
level
of
recep-
tor
crosstalk,
cAMP
activation,
and
AP1
signaling
(nontraditional
promoter
events),
and
as
these
differences
could
impact
on
the
activity
of
endocrine
disruptors
in
different
species,
this
area
warrants
further
exploration.
In
particular,
ligand-independent
activation
is
facilitated
by
low
levels
of
hormones
("prim-
ing
the
pump"),
suggesting
that
these
non-
traditional
means
of
receptor
activation
may
be
particularly
relevant
for
low-dose
exposures.
Gene
Transription
In
terms
of
specific
gene
transcription,
the
work
group
identified
a
need
to
assess
the
available
literature
on
gene
transcription
in
different
species
in
response
to
steroid
hor-
mones.
This
discussion
led
further
to a
con-
sensus
that
a
hormone-responsive
gene
chip
would
be
very
useful
for
making
this
assess-
ment.
Such
a
chip,
containing
a
battery
of
hormone-responsive
genes,
could
be
used
to
quantitate
changes
in
the
expression
of
these
genes
in
different
species
in
response
to
This
report
was
developed
at
the
Workshop
on
Characterizing
the
Effects
of
Endocrine
Disruptors
on
Human
Health
at
Environmental
Exposure
Levels
held
1
1-13
May
1998
in
Raleigh,
North
Carolina.
Address
correspondence
to
C.
Walker,
University
of
Texas,
MD
Anderson
Cancer
Center,
Science
Park
Research
Division,
Park
Road
1
C,
Smithville,
TX
78957.
Telephone:
(512)
237-2403.
Fax:
(512)
237-2475.
E-mail:
Received
25
September
1998;
accepted
27
May
1999.
Environmental
Health
Perspectives
*
Vol
107,
Supplement
4
*
August
1999
619