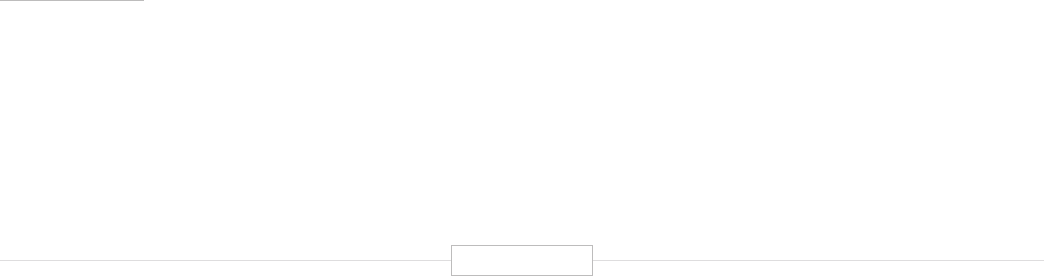
27
has occurred, whereby the speed increases and cost
decreases in sequencing have facilitated the assembly
of exabytes
48
of genomic information that can be
mined (assisted by highly advanced and automated
analytical systems) for unique insights into genome
structure and function.
49
As highlighted by Green:
Leading the signature advances has been
a greater than one million-fold reduction in
the cost of DNA sequencing. This decrease
has allowed the generation of innumerable
genome sequences, including hundreds of
thousands of human genome sequences (both
in research and clinical settings), and the
continuous development of assays to identify
and characterize functional genomic elements.
These new tools, together with increasingly
sophisticated statistical and computational
methods, have enabled researchers to create
rich catalogs of human genomic variants,
to gain an ever deepening understanding
of the functional complexities of the human
genome, and to determine the genomic
bases of thousands of human diseases.
50
This leads us to the first functional impact domain of
genetics and genomics in human medicine, minable
big data, and what it enables, discovery science.
1. Minable Big Data (Discovery Science)
Laurence Hurst highlights the central role
that data are playing in advancing function-
al applications of genomics, noting that:
Genomics is in an age of exploration and
discovery. Whether we are discovering the
genomes of more species, the genomes
of more individuals in a species, or more
genomes within an individual (at single-cell
An exabyte = bytes (,,,,,, bytes).
Stephens, Zachary D., et al. “Big Data: Astronomical or Genomical?” PLOS Biology, vol. , July . doi:./journal.pbio..
Green, Eric D., et al. “Perspective: Strategic Vision for Improving Human Health at the Forefront of Genomics.” Nature, vol. , Oct. .
Cheifet, Barbara. “Editorial: Where is genomics going next?” Genome Biology, vol. , no. , Jan. . doi:./s---.
Zielinski, Dina and Janiv Erlich. “Genetic Privacy in the Post-Covid Word.” Science, vol. , no. , Feb. .
Birney, Ewan. “Luminaries Share Their Thoughts on Advances in ‘Omics Over the Past Five Years.” Clinical Omics Magazine, vol. , no. , March-
April .
National Human Genome Research Institute. “Genetics vs. Genomics Fact Sheet.” www.genome.gov/about-genomics/fact-sheets/Genetics-vs-
Genomics#:~:text=All%human% beings%are%.,about%the%causes%of%diseases. Accessed May .
resolution), we are very much in a phase
where we are letting the data lead.
51
Studying one person’s genome (or in the case of the
“reference” human genome, a composite of a few peo-
ple) has provided valuable information regarding the
structure of the human genome and the number of
protein-coding genes. It has also allowed for compar-
ison to an expanding library of reference genomes for
other organisms, helping to identify regions of similar-
ity and difference that could help illuminate function-
ality. At a time when whole-genome sequencing cost
many millions of dollars per genome, the field was
limited to small volumes of sequenced genomes to
work with. As sequencing costs declined and sequenc-
ing speed increased, the ability to generate data from
a large number of individuals started to become real-
istic, and this has opened new horizons for research.
Currently, whole genome sequencing and whole
exome (the part of the genome formed from exons
that code proteins) sequencing is fast and affordable
(requiring just a day and $689 for whole genome
sequencing), and as affordability and speed have
increased, the number of sequenced individuals
has expanded exponentially. In a recent edition of
Science, it is noted that “today, more than 30 million
individuals have access to their detailed genomic
datasets,”
52
while Ewan Birney of the European
Bioinformatics Institute notes that “estimates show
that over 60 million patients will have their genome
sequenced in a healthcare context by 2025.”
53
Having access to an extremely large volume of se-
quenced individuals creates a dramatically enlarged
platform for discovery. Each of us has a unique
genome. While 99.9% of the genome between
individuals will be the same,
54
the 0.1% differenc-
es can add up to profoundly dissimilar physical
characteristics and differential predisposition to