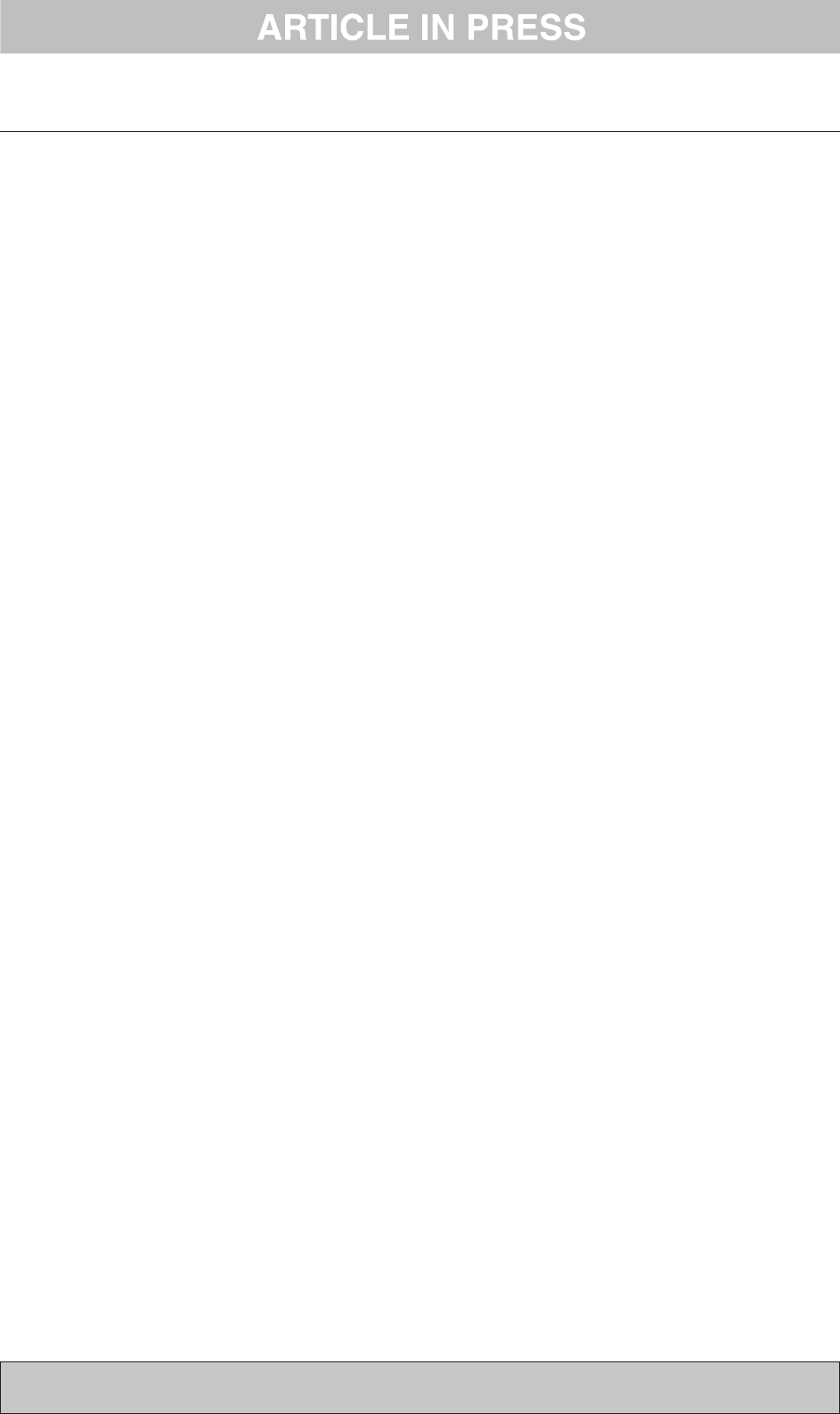
[56] A.E. Friedland, Y.B. Tzur, K.M. Esvelt, M.P. Colaiacovo, G.M.
Church, J.A. Calarco, Heritable genome editing in C. elegans
via a CRISPR–Cas9 system, Nat. Methods 10 (2013)
741–743.
[57] U. Irion, J. Krauss, C. Nusslein-Volhard, Precise and
efficient genome editing in zebrafish using the CRISPR/
Cas9 system, Development 141 (2014) 4827–4830.
[58] Z. Feng, B. Zhang, W. Ding, X. Liu, D.L. Yang, P. Wei, et al.,
Efficient genome editing in plants using a CRISPR/Cas
system, Cell Res. 23 (2013) 1229–1232.
[59] T. Hai, F. Teng, R. Guo, W. Li, Q. Zhou, One- step
generation of knockout pigs by zygote injection of
CRISPR/Cas system, Cell Res. 24 (2014) 372–375.
[60] M. Ikeda, S. Matsuyama, S. Akagi, K. Ohkoshi, S.
Nakamura, S. Minabe, et al., Correction of a disease
mutation using CRISPR/Cas9-assisted genome editing in
Japanese black cattle, Sci. Rep. 7 (2017), 17827.
[61] E. Zuo, Y.J. Cai, K. Li, Y. Wei, B.A. Wang, Y. Sun, et al.,
One-step generation of complete gene knockout mice and
monkeys by CRISPR/Cas9-mediated gene editing with
multiple sgRNAs, Cell Res. 27 (2017) 933–945.
[62] H. Ma, N. Marti-Gutierrez, S.W. Park, J. Wu, Y. Lee, K.
Suzuki, et al., Correction of a pathogenic gene mutation in
human embryos, Nature 548 (2017) 413–419.
[63] X. Kang, W. He, Y. Huang, Q. Yu, Y. Chen, X. Gao, et al.,
Introducing precise genetic modifications into human 3PN
embryos by CRISPR/Cas-mediated genome editing, J. Assist.
Reprod. Genet. 33 (2016) 581–588.
[64] T. Gaj, C.A. Gersbach, C.F. Barbas III, ZFN, TALEN, and
CRISPR/Cas-based methods for genome engineering,
Trends Biotechnol. 31 (2013) 397–405.
[65] T. Nakamura, A.R. Gehrke, J. Lemberg, J. Szymaszek, N.H.
Shubin, Digits and fin rays share common developmental
histories, Nature 537 (2016) 225–228.
[66] J.J. Russell, J.A. Theriot, P. Sood, W.F. Marshall, L.F.
Landweber, L. Fritz-Laylin, et al., Non-model model
organisms, BMC Biol. 15 (2017) 55.
[67] W. Shang, F. Wang, G. Fan, H. Wang, Key elements for
designing and performing a CRISPR/Cas9-based genetic
screen, J. Genet. Genomics 44 (2017) 439–449.
[68] H.K. Liao, F. Hatanaka, T. Araoka, P. Reddy, M.Z. Wu, Y.
Sui, et al., In vivo target gene activation via CRISPR/Cas9-
Mmdiated trans-epigenetic modulation, Cell 171 (2017)
(1495-507.e15).
[69] M. Yang, L. Zhang, J. Stevens, G. Gibson, CRISPR/Cas9
mediated generation of stable chondrocyte cell lines with
targeted gene knockouts; analysis of an aggrecan knockout
cell line, Bone 69 (2014) 118–125.
[70] X.J. Lu, Y.Y. Xiang, X.J. Li, CRISPR screen: a high-
throughput approach for cancer genetic research, Clin.
Genet. 88 (2015) 32–33.
[71] E. Charpentier, H. Richter, J. van der Oost, M.F. White,
Biogenesis pathways of RNA guides in archaeal and
bacterial CRISPR–Cas adaptive immunity, FEMS Microbiol.
Rev. 39 (2015) 428–441.
[72] T.S. Klann, J.B. Black, M. Chellappan, A. Safi, L. Song, I.B.
Hilton, et al., CRISPR–Cas9 epigenome editing enables
high-throughput screening for functional regulatory ele-
ments in the human genome, Nat. Biotechnol. 35 (2017)
561
–568.
[73] A. Mazo-Vargas, C. Concha, L. Livraghi, D. Massardo, R.W.
R. Wallbank, L. Zhang, et al., Macroevolutionary shifts of
WntA function potentiate butterfly wing-pattern diversity,
Proc. Natl. Acad. Sci. U. S. A. 114 (2017) 10701–10706.
[74] P. Atanes, I. Ruz-Maldonado, R. Hawkes, B. Liu, S.J.
Persaud, S. Amisten, Identifying signall ing pathways
regulated by GPRC5B in beta-cells by CRISPR–Cas9-
mediated genome editing, Cell. Physiol. Biochem. 45 (2018)
656–666.
[75] S. Chi, A. Weiss, H.A. Wang, CRISPR-based toolbox for
studying T cell signal transduction, Biomed. Res. Int. 2016
(2016), 5052369.
[76] C. Kasap, O. Elemento, T.M. Kapoor, DrugTargetSeqR: a
genomics- and CRISPR–Cas9-based method to analyze
drug targets, Nat. Chem. Biol. 10 (2014) 626–628.
[77] B.E. Housden, A.J. Valvezan, C. Kelley, R. Sopko, Y. Hu, C.
Roesel, et al., Identification of potential drug targets for
tuberous sclerosis complex by synthetic screens combining
CRISPR-based knockouts with RNAi, Sci. Signal. 8 (2015) rs9.
[78] I. Hyun, A. Wilkerson, J. Johnston, Embryology policy:
revisit the 14-day rule, Nature 533 (7602) (2016) 169–171.
[79] Human embryo culture: discussions concerning the statu-
tory time limit for maintaining human embryos in culture in
light of some recent scientific developments. Nuffield
Council on Bioethics, 2017 [cited 2018 May 29]. Available
from: http://nuffi eldbioethics.org/wp-content/uploads/
Human-Embryo-Culture-web-FINAL.pdf.
[80] R.A. Charo, The hunting of the snark: the moral status of
embryos, right-to-lifers, and Third World women, Stanf. Law
Pol. Rev. 6 (1995) 11–37.
[81] B. Wennergr en, Hum an ri ghts of an em bryo , J. Int .
Bioethique 2 (1991) 46–49.
[82] J.J. Miklavcic, P. Flaman, Personhood status of the human
zygote, embryo, fetus, Linacre Q. 84 (2017) 130–144.
[83] J.W. McDonald, X.Z. Liu, Y. Qu, S. Liu, S.K. Mickey, D.
Turetsky, et al., Transplanted embryonic stem cells survive,
differentiate and promote recovery in injured rat spinal cord,
Nat. Med. 5 (1999) 1410–1412.
[84] L.M. Bjorklund, R. Sanchez-Pernaute, S. Chung, T.
Andersson, I.Y. Chen, K.S. McNaught, et al., Embryonic
stem cells develop into functional dopaminergic neurons
after transplantation in a Parkinson rat model, Proc. Natl.
Acad. Sci. U. S. A. 99 (2002) 2344–2349.
[85] V.F. Hamrahi, J. Goverman, W. Jung, J.C. Wu, A.J.
Fischman, R.G. Tompkins, et al., In vivo molecular imaging
of murine embryonic stem cells delivered to a burn wound
surface via Integra(R) scaffolding, J. Burn Care Res. 33
(2012) e49–e54.
[86] D.K. Singla, A. Ahmed, R. Singla, B. Yan, Embryonic stem
cells improve cardiac function in doxorubicin-induced
cardiomyopathy mediated through multiple mechanisms,
Cell Transplant. 21 (2012) 1919–1930.
[87] F.P. Busardo, M. Gulino, S. Napoletano, S. Zaami, P. Frati,
The evolution of legislation in the field of medically assisted
reproduction and embryo stem cell research in European
union members, Biomed. Res. Int. 2014 (2014), 307160.
[88] R. Dajani, Jordan's stem-cell law can guide the Middle East,
Nature 510 (2014) 189.
[89] R.T. Manguso, H.W. Pope, M.D. Zimmer, F.D. Brown, K.B.
Yates, B.C. Miller, et al., In vivo CRISPR screening
identifies Ptpn2 as a cancer immunotherapy target, Nature
547 (2017) 413–418.
[90] J. Drost, R. van Boxtel, F. Blokzijl, T. Mizutani, N. Sasaki, V.
Sasselli, et al., Use of CRISPR-modified human stem cell
organoids to study the origin of mutational signatures in
cancer, Science 358 (2017) 234–
238.
[91] J. Chu, G. Galicia-Vazquez, R. Cencic, J.R. Mills, A.
Katigbak, J.A. Porco Jr., et al., CRISPR-mediated drug-
11Review: CRISPR Ethics
Please cite this article as: C. Brokowski, M. Adli, CRISPR Ethics: Moral Considerations for Applications of a Powerful Tool, J. Mol. Biol.
(2018), https://doi.org/10.1016/j.jmb.2018.05.044