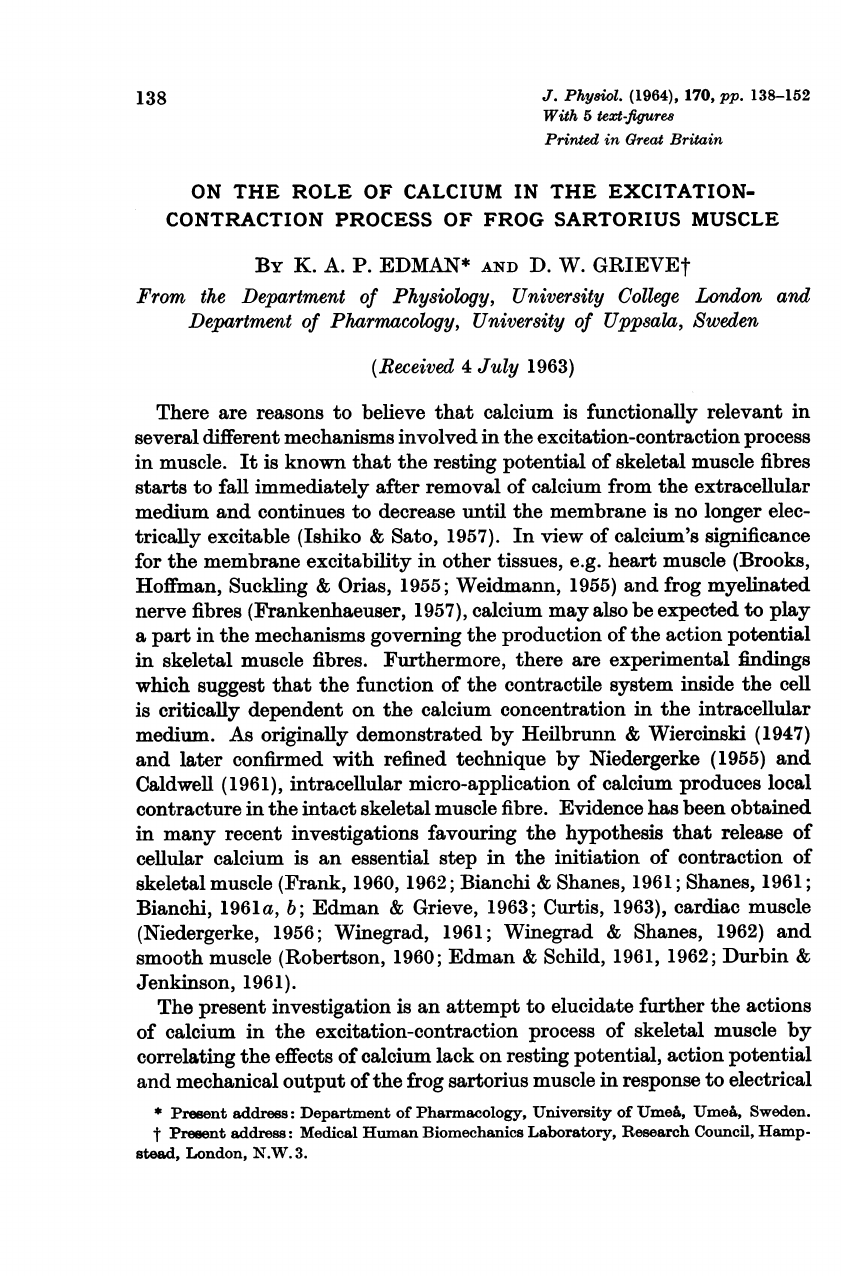
138
J.
Physiol.
(1964),
170,
pp.
138-152
With
5
text-figure8
Printed
in
Great
Britain
ON
THE
ROLE
OF
CALCIUM
IN
THE
EXCITATION-
CONTRACTION
PROCESS
OF
FROG
SARTORIUS
MUSCLE
BY
K.
A.
P.
EDMAN*
AND
D.
W.
GRIEVEt
From
the
Department
of
Physiology,
University
College
London
and
Department
of
Pharmacology,
University
of
Uppsala,
Sweden
(Received
4
July
1963)
There
are
reasons
to
believe
that
calcium
is
functionally
relevant
in
several
different
mechanisms
involved
in
the
excitation-contraction
process
in
muscle.
It
is
known
that
the
resting
potential
of
skeletal
muscle
fibres
starts
to
fall
immediately
after
removal
of
calcium
from
the
extracellular
medium
and
continues
to
decrease
until
the
membrane
is
no
longer
elec-
trically
excitable
(Ishiko
&
Sato,
1957).
In
view
of
calcium's
significance
for
the
membrane
excitability
in
other
tissues,
e.g.
heart
muscle
(Brooks,
Hoffman,
Suckling
&
Orias,
1955;
Weidmann,
1955)
and
frog
myelinated
nerve
fibres
(Frankenhaeuser,
1957),
calcium
may
also
be
expected
to
play
a
part
in
the
mechanisms
governing
the
production
of
the
action
potential
in
skeletal
muscle
fibres.
Furthermore,
there
are
experimental
findings
which
suggest
that
the
function
of
the
contractile
system
inside
the
cell
is
critically
dependent
on
the
calcium
concentration
in
the
intracellular
medium.
As
originally
demonstrated
by
Heilbrunn
&
Wiercinski
(1947)
and
later
confirmed
with
refined
technique
by
Niedergerke
(1955)
and
Caldwell
(1961),
intracellular
micro-application
of
calcium
produces
local
contracture
in
the
intact
skeletal
muscle
fibre.
Evidence
has
been
obtained
in
many
recent
investigations
favouring
the
hypothesis
that
release
of
cellular
calcium
is
an
essential
step
in
the
initiation
of
contraction
of
skeletal
muscle
(Frank,
1960,
1962;
Bianchi
&
Shanes,
1961;
Shanes,
1961;
Bianchi,
1961a,
b;
Edman
&
Grieve,
1963;
Curtis,
1963),
cardiac
muscle
(Niedergerke,
1956;
Winegrad,
1961;
Winegrad
&
Shanes,
1962)
and
smooth
muscle
(Robertson,
1960;
Edman
&
Schild,
1961,
1962;
Durbin
&
Jenkinson,
1961).
The
present
investigation
is
an
attempt
to
elucidate
further
the
actions
of
calcium
in
the
excitation-contraction
process
of
skeletal
muscle
by
correlating
the
effects
of
calcium
lack
on
resting
potential,
action
potential
and
mechanical
output
of
the
frog
sartorius
muscle
in
response
to
electrical
*
Present
address:
Department
of
Pharmacology,
University
of
Umea,
Umea,
Sweden.
t
Present
address:
Medical
Human
Biomechanics
Laboratory,
Research
Council,
Hamp-
stead,
London,
N.W.
3.
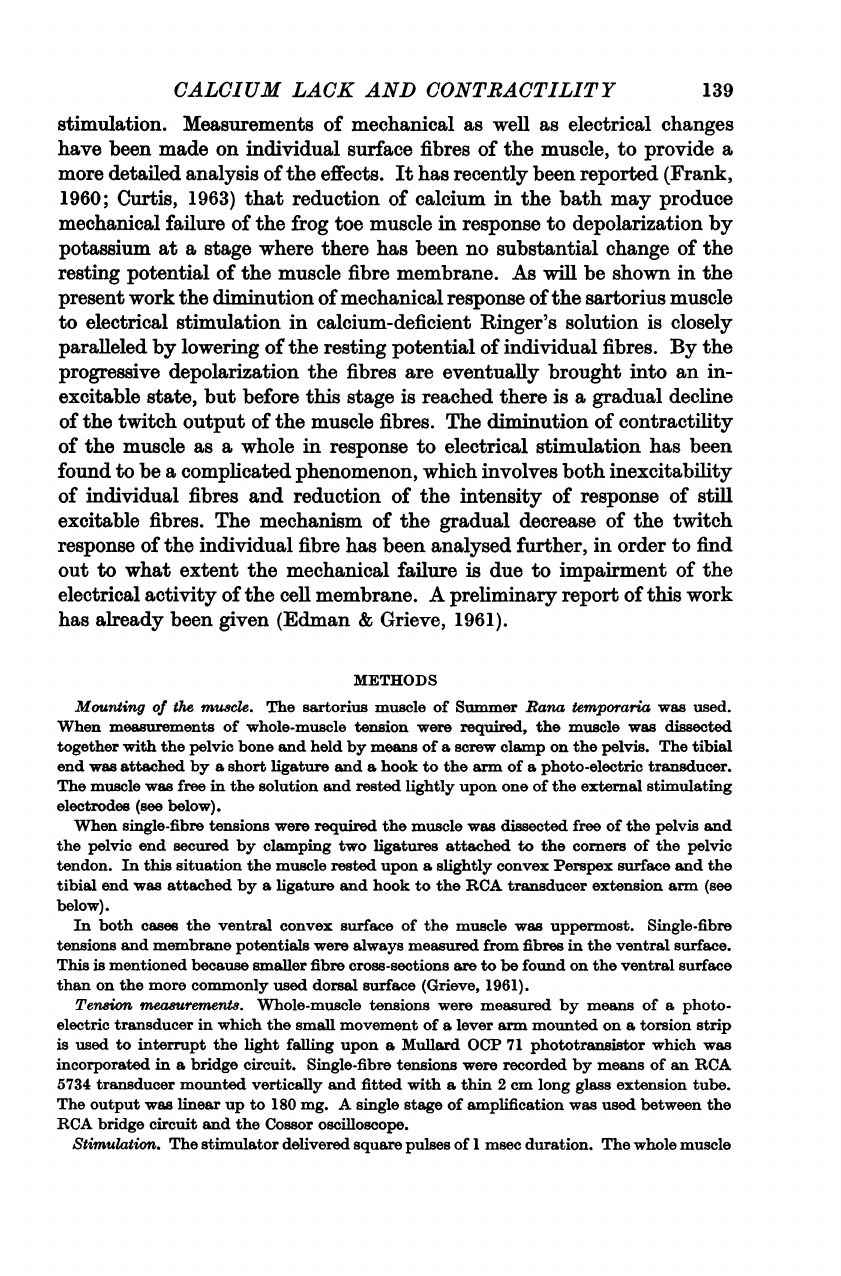
CALCIUM
LACK
AND
CONTRACTILITY
139
stimulation.
Measurements
of
mechanical
as
well
as
electrical
changes
have
been
made
on
individual
surface
fibres
of
the
muscle,
to
provide
a
more
detailed
analysis
of
the
effects.
It
has
recently
been
reported
(Frank,
1960;
Curtis,
1963)
that
reduction
of
calcium
in
the
bath
may
produce
mechanical
failure
of
the
frog
toe
muscle
in
response
to
depolarization
by
potassium
at
a
stage
where
there
has
been
no
substantial
change
of
the
resting
potential
of
the
muscle
fibre
membrane.
As
will
be
shown
in
the
present
work
the
diminution
of
mechanical
response
of
the
sartorius
muscle
to
electrical
stimulation
in
calcium-deficient
Ringer's
solution
is
closely
paralleled
by
lowering
of
the
resting
potential
of
individual
fibres.
By
the
progresive
depolarization
the
fibres
are
eventually
brought
into
an
in-
excitable
state,
but
before
this
stage
is
reached
there
is
a
gradual
decline
of
the
twitch
output
of
the
muscle
fibres.
The
diminution
of
contractility
of
the
muscle
as
a
whole
in
response
to
electrical
stimulation
has
been
found
to
be
a
complicated
phenomenon,
which
involves
both
inexcitability
of
individual
fibres
and
reduction
of
the
intensity
of
response
of
still
excitable
fibres.
The
mechanism
of
the
gradual
decrease
of
the
twitch
response
of
the
individual
fibre
has
been
analysed
further,
in
order
to
find
out
to
what
extent
the
mechanical
failure
is
due
to
impairment
of
the
electrical
activity
of
the
cell
membrane.
A
preliminary
report
of
this
work
has
already
been
given
(Edman
&
Grieve,
1961).
METHODS
Mounting
of
the
muscle.
The
sartorius
muscle
of
Summer
Rana
temporaria
was
used.
When
measurements
of
whole-muscle
tension
were
required,
the
muscle
was
dissected
together
with
the
pelvic
bone
and
held
by
means
of
a
screw
clamp
on
the
pelvis.
The
tibial
end
was
attached
by
a
short
ligature
and
a
hook
to
the
arm
of
a
photo-electric
transducer.
The
muscle
was
free
in
the
solution
and
rested
lightly
upon
one
of
the
external
stimulating
electrodes
(see
below).
When
single-fibre
tensions
were
required
the
muscle
was
dissected
free
of
the
pelvis
and
the
pelvic
end
secured
by
clamping
two
ligatures
attached
to
the
comers
of
the
pelvic
tendon.
In
this
situation
the
muscle
rested
upon
a
slightly
convex
Perspex
surface
and
the
tibial
end
was
attached
by
a
ligature
and
hook
to
the
RCA
transducer
extension
arm
(see
below).
In
both
cases
the
ventral
convex
surface
of
the
muscle
was
uppermost.
Single-fibre
tensions
and
membrane
potentials
were
always
measured
from
fibres
in
the
ventral
surface.
This
is
mentioned
because
smaller
fibre
cross-sections
are
to
be
found
on
the
ventral
surface
than
on
the
more
commonly
used
dorsal
surface
(Grieve,
1961).
Tension
measurements.
Whole-muscle
tensions
were
measured
by
means
of
a
photo-
electric
transducer
in
which
the
small
movement
of
a
lever
arm
mounted
on
a
torsion
strip
is
used
to
interrupt
the
light
falling
upon
a
Mullard
OCP
71 phototransistor
which
was
incorporated
in
a
bridge
circuit.
Single-fibre
tensions
were
recorded
by
means
of
an
RCA
5734
transducer
mounted
vertically
and
fitted
with
a
thin
2
cm
long
glass
extension
tube.
The
output
was
linear
up
to
180
mg.
A
single
stage
of
amplification
was
used
between
the
RCA
bridge
circuit
and
the
Cossor
oscilloscope.
Stimulation.
The
stimulator
delivered
square
pulses
of
1
msec
duration.
The
whole
muscle
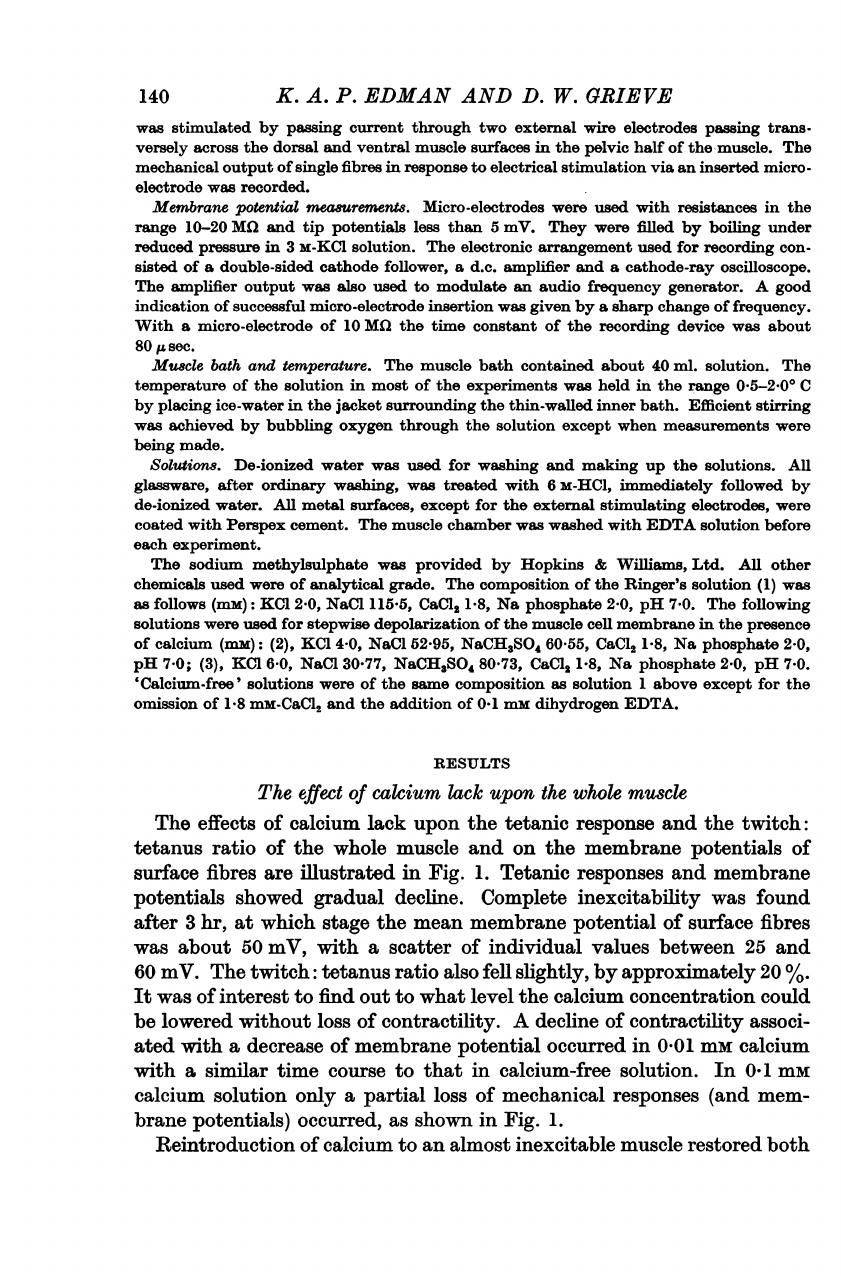
140
K.
A.
P.
EDMAN
AND
D.
W.
GRIEVE
was
stimulated
by
passing
current
through
two
external
wire
electrodes
passing
trans-
versely
across
the
dorsal
and
ventral
muscle
surfaces
in
the
pelvic
half
of
the
muscle.
The
mechanical
output
of
single
fibres
in
response
to
electrical
stimulation
via
an
inserted
micro-
electrode
was
recorded.
Membrane
potential
meaeuremente.
Micro-electrodes
were
used
with
resistances
in
the
range
10-20
MQ
and
tip
potentials
less
than
5
mV.
They
were
filled
by
boiling
under
reduced
pressure
in 3
x-KC1
solution.
The
electronic
arrangement
used
for
recording
con-
sisted
of
a
double-sided
cathode
follower,
a
d.c.
amplifier
and
a
cathode-ray
oscilloscope.
The
amplifier
output
was
also
used
to
modulate
an
audio
frequency
generator.
A
good
indication
of
successful
micro-electrode
insertion
was
given
by
a
sharp
change
of
frequency.
With
a
micro-electrode
of
10
MCI
the
time
constant
of
the
recording
device
was
about
80
!4sec.
Mwucle
bath
and
temperature.
The
muscle
bath
contained
about
40
ml.
solution.
The
temperature
of
the
solution
in
most
of
the
experiments
was
held
in
the
range
0.5-2.0o
C
by
placing
ice-water
in
the
jacket
surrounding
the
thin-walled
inner
bath.
Efficient
stirring
was
achieved
by
bubbling
oxygen
through
the
solution
except
when
measurements
were
being
made.
Solution&.
De-ionized
water
was
used
for
washing
and
making
up
the
solutions.
All
glassware,
after
ordinary
washing,
was
treated
with
6
x-HCI,
immediately
followed
by
de-ionized
water.
All
metal
surfaces,
except
for
the
external
stimulating
electrodes,
were
coated
with
Perspex
cement.
The
muscle
chamber
was
washed
with
EDTA
solution
before
each
experiment.
The
sodium
methylsulphate
was
provided
by
Hopkins
&
Williams,
Ltd.
All
other
chemicals
used
were
of
analytical
grade.
The
composition
of the
Ringer's
solution
(1)
was
as
follows
(mx):
KlC
2-0,
NaCl
115-5,
CaCl2
1-8,
Na
phosphate
2-0,
pH
7-0.
The
following
solutions
were
used
for
stepwise
depolarization
of
the
muscle
cell
membrane
in
the
presence
of
calcium
(mm):
(2),
KC1
4-0,
NaCl
52-95,
NaCH3SO4
60-55,
CaCl2
1-8,
Na
phosphate
2-0,
pH
7-0;
(3),
KCI
6-0,
NaCl
30-77,
NaCH3SO4
80-73,
CaCl2
1-8,
Na
phosphate
2-0,
pH
7-0.
'Calcium-free'
solutions
were
of
the
same
composition
as
solution
1
above
except
for
the
omission
of
1-8
m
-CaCl2
and
the
addition
of
0-1
mm
dihydrogen
EDTA.
RESULTS
The
effect
of
calcium
lack
upon
the
whole
muscle
The
effects
of
calcium
lack
upon
the
tetanic
response
and
the
twitch:
tetanus
ratio
of
the
whole
muscle
and
on
the
membrane
potentials
of
surface
fibres
are
illustrated
in
Fig.
1.
Tetanic
responses
and
membrane
potentials
showed
gradual
decline.
Complete
inexcitability
was
found
after
3
hr,
at
which
stage
the
mean
membrane
potential
of
surface
fibres
was
about
50
mV,
with
a
scatter
of
individual
values
between
25
and
60
mV.
The
twitch:
tetanus
ratio
also
fell
slightly,
by
approximately
20
%.
It
was
of
interest
to
find
out
to
what
level
the
calcium
concentration
could
be
lowered
without
loss
of
contractility.
A
decline
of
contractility
associ-
ated
with
a
decrease
of
membrane
potential
occurred
in
0-01
mm
calcium
with
a
similar
time
course
to
that
in
calcium-free
solution.
In
0-1
mm
calcium
solution
only
a
partial
loss
of
mechanical
responses
(and
mem-
brane
potentials)
occurred,
as
shown
in
Fig.
1.
Reintroduction
of
calcium
to
an
almost
inexcitable
muscle
restored
both
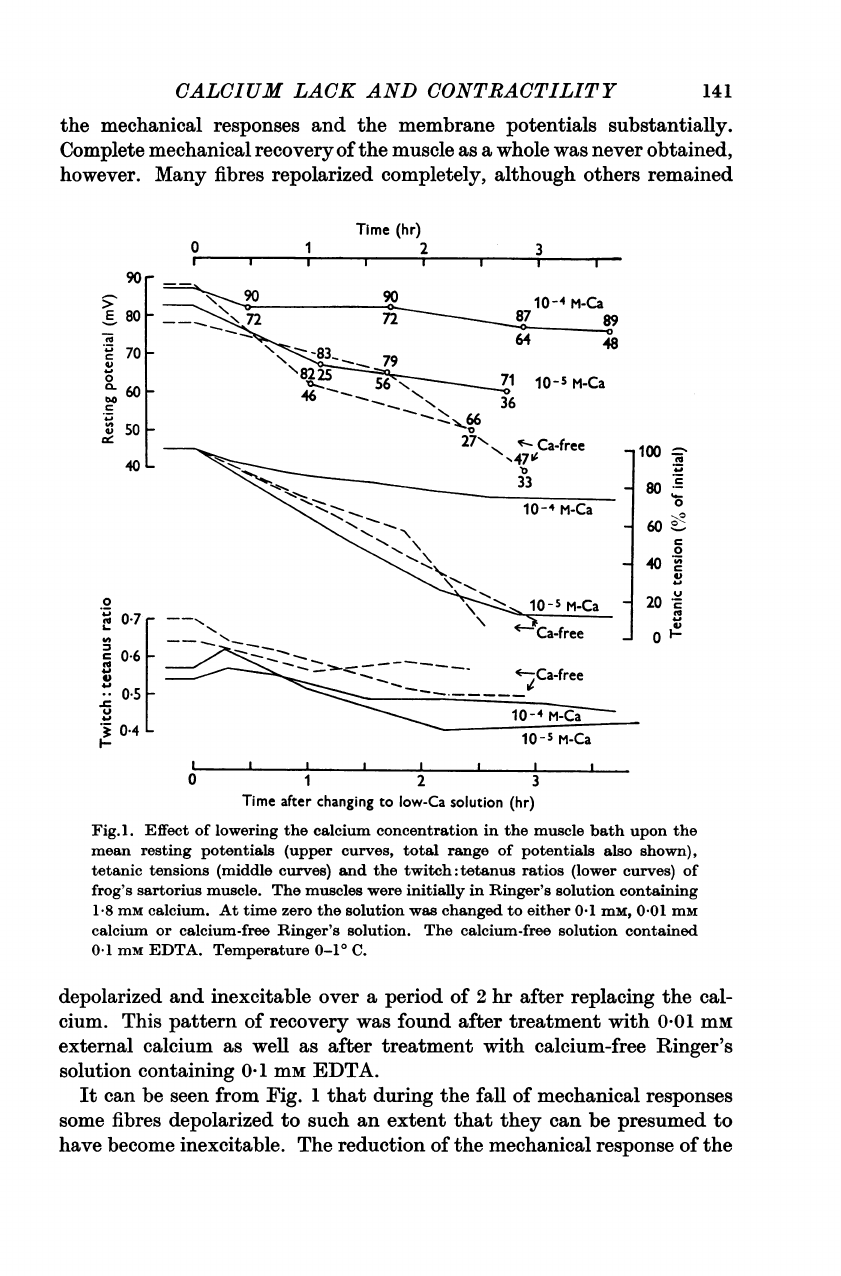
CALCIUM
LACK
AND
CONTRACTILITY
the
mechanical
responses
and
the
membrane
potentials
substantially.
Complete
mechanical
recovery
of
the
muscle
as
a
whole
was
never
obtained,
however.
Many
fibres
repolarized
completely,
although
others
remained
90r
E80
a
70
0
0-60
vi50
40
0
to
._
C
(4
4,
._
Ut
0
r
Time
(hr)
1
2
1
1
0-7
0-6
F
05
04
3
100
=,
80
:
0
-0
60
2
20
20
C
o6
(-Ca-free
_-
__
_
10-4
M-Ca
10-5
M-Ca
I
I
I
I I
I
0
1
2
3
Time
after
changing
to
low-Ca
solution
(hr)
Fig.l.
Effect
of
lowering
the
calcium
concentration
in
the
muscle
bath
upon
the
mean
resting
potentials
(upper
curves,
total
range
of
potentials
also
shown),
tetanic
tensions
(middle
curves)
and
the
twitch:tetanus
ratios
(lower
curves)
of
frog's
sartorius
muscle.
The
muscles
were
initially
in
Ringer's
solution
containing
1-8
mM
calcium.
At
time
zero
the
solution
was
changed
to
either
0-1
mM,
0-01
mM
calcium
or
calcium-free
Ringer's
solution.
The
calcium-free
solution
contained
0-1
mM
EDTA.
Temperature
0-1°
C.
depolarized
and
inexcitable
over
a
period
of
2
hr
after
replacing
the
cal-
cium.
This
pattern
of
recovery
was
found
after
treatment
with
0-01
mM
extemal
calcium
as
well
as
after
treatment
with
calcium-free
Ringer's
solution
containing
0-1
mm
EDTA.
It
can
be
seen
from
Fig.
1
that
during
the
fall
of
mechanical
responses
some
fibres
depolarized
to
such
an
extent
that
they
can
be
presumed
to
have
become
inexcitable.
The
reduction
of
the
mechanical
response
of
the
141
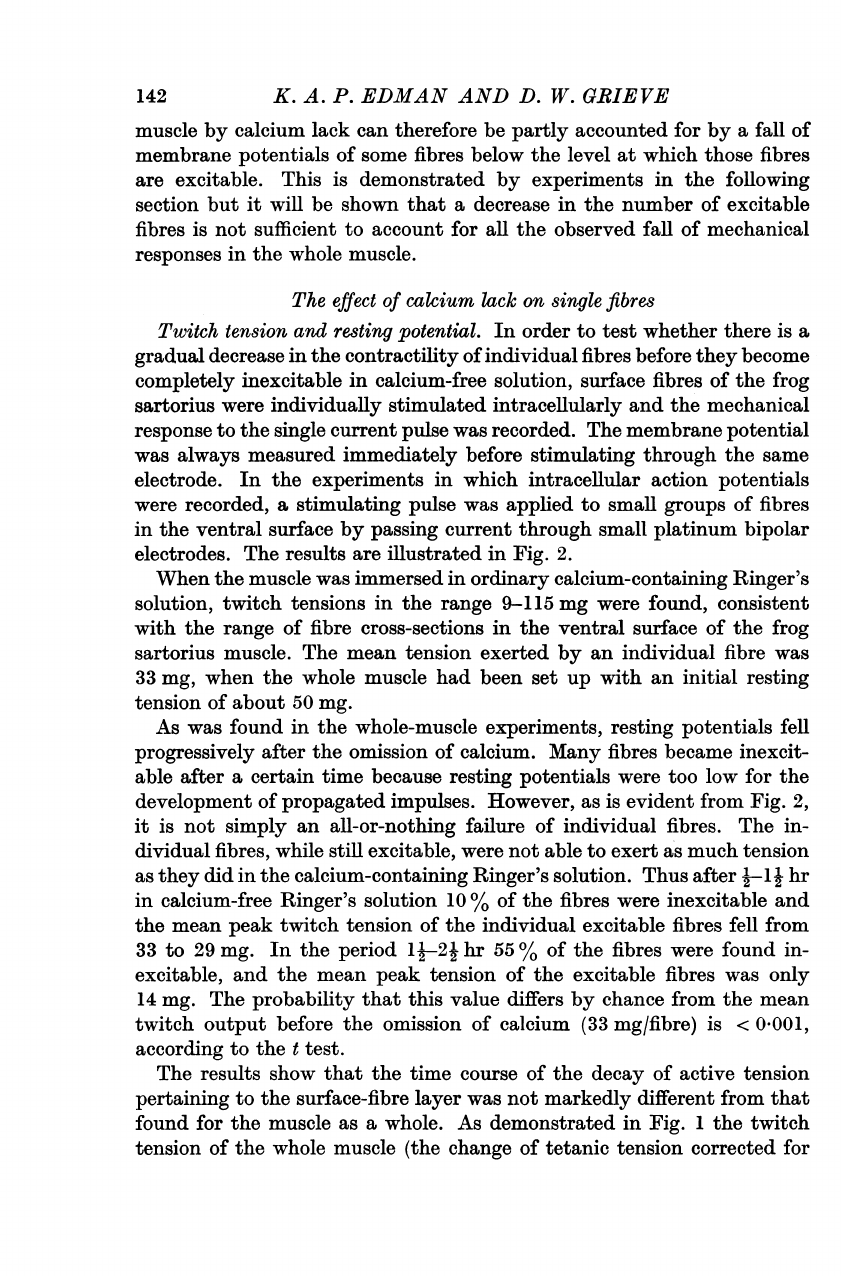
K.
A.
P.
EDMAN
AND
D.
W.
GRIEVE
muscle
by
calcium
lack
can
therefore
be
partly
accounted
for
by
a
fall
of
membrane
potentials
of
some
fibres
below
the
level
at
which
those
fibres
are
excitable.
This
is
demonstrated
by
experiments
in
the
following
section
but
it
will
be
shown
that
a
decrease
in
the
number
of
excitable
fibres
is
not
sufficient
to
account
for
all
the
observed
fall
of
mechanical
responses
in
the
whole
muscle.
The
effect
of
calcium
lack
on
single
fibres
Twitch
tension
and
resting
potential.
In
order
to
test
whether
there
is
a
gradual
decrease
in
the
contractility
of
individual
fibres
before
they
become
completely
inexcitable
in
calcium-free
solution,
surface
fibres
of
the
frog
sartorius
were
individually
stimulated
intracellularly
and
the
mechanical
response
to
the
single
current
pulse
was
recorded.
The
membrane
potential
was
always
measured
immediately
before
stimulating
through
the
same
electrode.
In
the
experiments
in
which
intracellular
action
potentials
were
recorded,
a
stimulating
pulse
was
applied
to
small
groups
of
fibres
in
the
ventral
surface
by
passing
current
through
small
platinum
bipolar
electrodes.
The
results
are
illustrated
in
Fig.
2.
When
the
muscle
was
immersed
in
ordinary
calcium-containing
Ringer's
solution,
twitch
tensions
in
the
range
9-115
mg
were
found,
consistent
with
the
range
of
fibre
cross-sections
in
the
ventral
surface
of
the
frog
sartorius
muscle.
The
mean
tension
exerted
by
an
individual
fibre
was
33
mg,
when
the
whole
muscle
had
been
set
up
with
an
initial
resting
tension
of
about
50
mg.
As
was
found
in
the
whole-muscle
experiments,
resting
potentials
fell
progressively
after
the
omission
of
calcium.
Many
fibres
became
inexcit-
able
after
a
certain
time
because
resting
potentials
were
too
low
for
the
development
of
propagated
impulses.
However,
as
is
evident
from
Fig.
2,
it
is
not
simply
an
all-or-nothing
failure
of
individual
fibres.
The
in-
dividual
fibres,
while
still
excitable,
were
not
able
to
exert
as
much
tension
as
they
did
in
the
calcium-containing
Ringer's
solution.
Thus
after
j-1j
hr
in
calcium-free
Ringer's
solution
10
%
of
the
fibres
were
inexcitable
and
the
mean
peak
twitch
tension
of
the
individual
excitable
fibres
fell
from
33
to
29
mg.
In
the
period
1-
21
hr
55
%
of
the
fibres
were
found
in-
excitable,
and
the
mean
peak
tension
of
the
excitable
fibres
was
only
14
mg.
The
probability
that
this
value
differs
by
chance
from
the
mean
twitch
output
before
the
omission
of
calcium
(33
mg/fibre)
is
<
0-001,
according
to
the
t
test.
The
results
show
that
the
time
course
of
the
decay
of
active
tension
pertaining
to
the
surface-fibre
layer
was
not
markedly
different
from
that
found
for
the
muscle
as
a
whole.
As
demonstrated
in
Fig.
1
the
twitch
tension
of
the
whole
muscle
(the
change
of
tetanic
tension
corrected
for
142
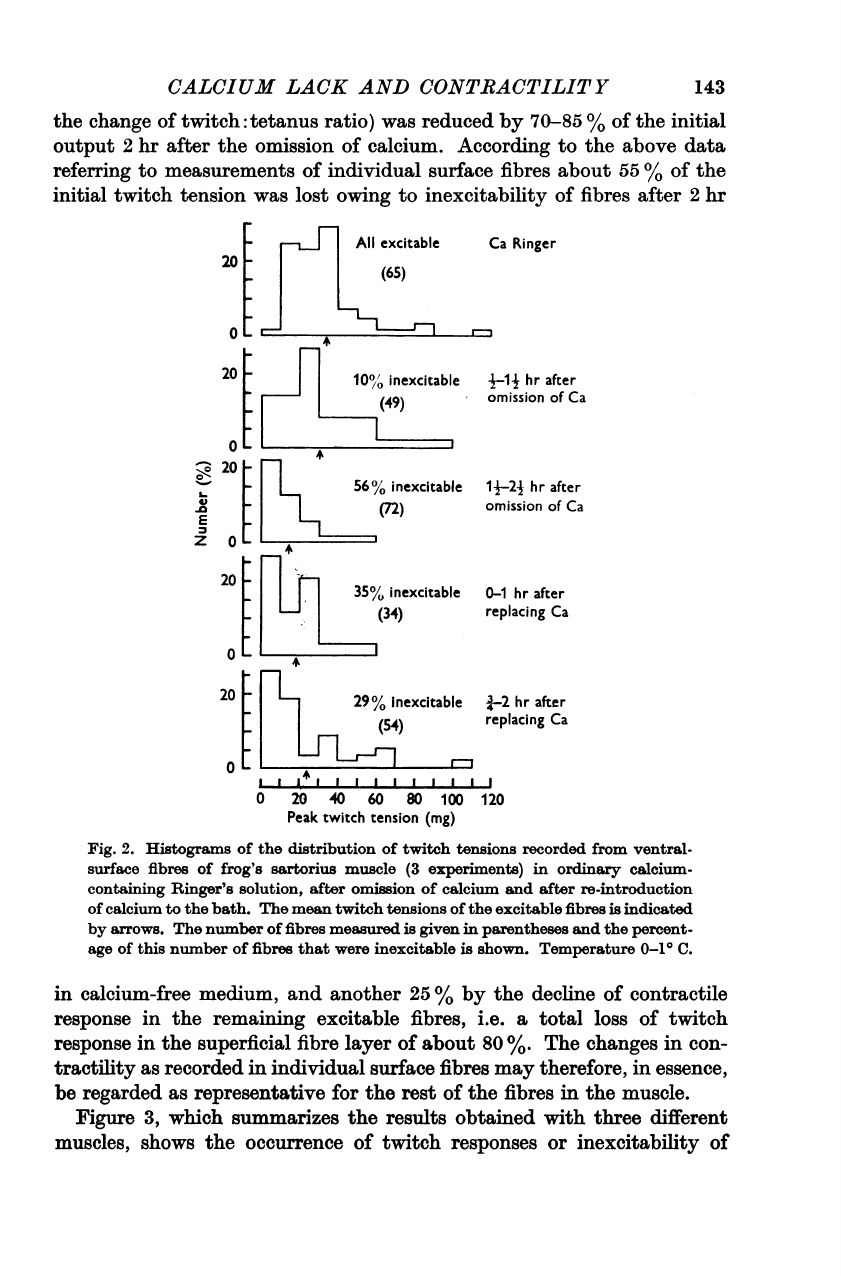
CALCIUM
LACK
AND
CONTRACTILITY
the
change
of
twitch:
tetanus
ratio)
was
reduced
by
70-85
%
of
the
initial
output
2
hr
after
the
omission
of
calcium.
According
to
the
above
data
referring
to
measurements
of
individual
surface
fibres
about
55
%
of
the
initial
twitch
tension
was
lost
owing
to
inexcitability
of
fibres
after
2
hr
01-
-
E
z
All
excitable
Ca
20
F
(65)
0L
20
[
100/'
inexcitable
4.-1
56%
inexcitable
1P:
2:
L
(72)
omi
4
L0
_
9
35%
inexcitable
0-1
(34)
repl
20
29%
inexcitable
4-2
°
-
t
l-,g'
~~~~~repl
I
I
11
11 11
1
111
0
20
40
60
80
100
120
Peak
twitch
tension
(mg)
Ringer
4I
hr
after
ission
of
Ca
24
hr
after
ssion
of
Ca
hr
after
acing
Ca
hr
after
acing
Ca
Fig.
2.
Histograms
of
the
distribution
of
twitch
tensions
recorded
from
ventral-
surface
fibres
of
frog's
sartorius
muscle
(3
experiments)
in
ordinary
calcium-
containing
Ringer's
solution,
after
omi#sion
of
calcium
and
after
re-introduction
of
calcium
to
the
bath.
The
mean
twitch
tensions
of
the
excitable
fibres
is
indicated
by
arrows.
The
number
of
fibres
measured
is
given
in
parentheses
and
the
percent-
age
of
this
number
of
fibres
that
were
inexcitable
is
shown.
Temperature
0-1°
C.
in
calcium-free
medium,
and
another
25
%
by
the
decline
of
contractile
response
in
the
remaining
excitable
fibres,
i.e.
a
total
loss
of
twitch
response
in
the
superficial
fibre
layer
of
about
80
%.
The
changes
in
con-
tractility
as
recorded
in
individual
surface
fibres
may
therefore,
in
essence,
be
regarded
as
representative
for
the
rest
of
the
fibres
in
the
muscle.
Figure
3,
which
summarizes
the
results
obtained
with
three
different
muscles,
shows
the
occurrence
of
twitch
responses
or
inexcitability
of
143
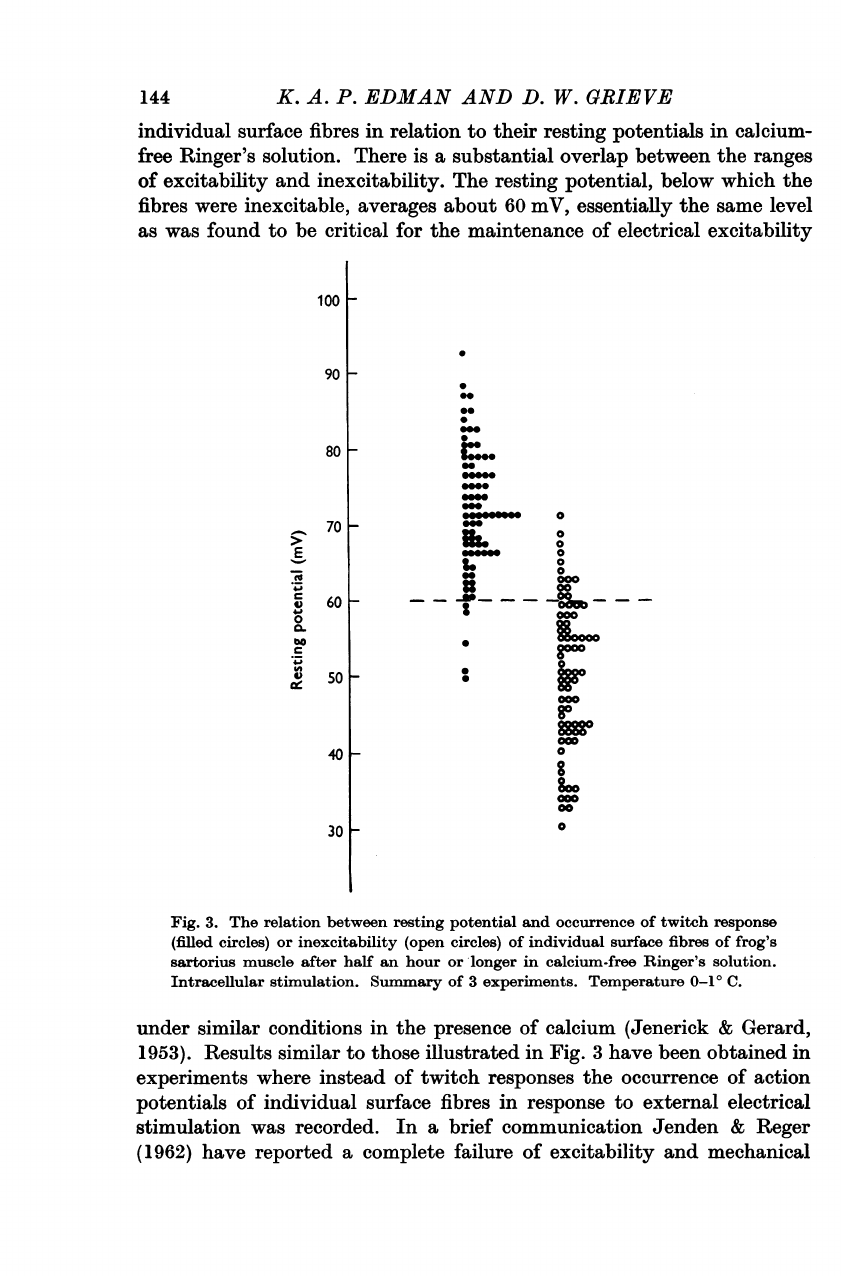
144
K.
A.
P.
EDMIAN
AND
D.
W.
GRIEVE
individual
surface
fibres
in
relation
to
their
resting
potentials
in
calcium-
free
Ringer's
solution.
There
is
a
substantial
overlap
between
the
ranges
of
excitability
and
inexcitability.
The
resting
potential,
below
which
the
fibres
were
inexcitable,
averages
about
60
mV,
essentially
the
same
level
as
was
found
to
be
critical
for
the
maintenance
of
electrical
excitability
100
F-
90
M-
80
F
70
F-
>
1-1
E
C
0
0.
be
C-
60
V
50
F
40F
30
F
0
*0
0
O"~~~
0
000"
00"
S
O
*6
0
mee
0
to
0
@00
@
000
800
=00
90
cooo
8
R
8000
00
0
Fig.
3.
The
relation
between
resting
potential
and
occurrence
of
twitch
response
(filled
circles)
or
inexcitability
(open
circles)
of
individual
surface
fibres
of
frog's
sartorius
muscle
after
half
an
hour
or
longer
in
calcium-free
Ringer's
solution.
Intracellular
stimulation.
Summary
of
3
experiments.
Temperature
0-1°
C.
under
similar
conditions
in
the
presence
of
calcium
(Jenerick
&
Gerard,
1953).
Results
similar
to
those
illustrated
in
Fig.
3
have
been
obtained
in
experiments
where
instead
of
twitch
responses
the
occurrence
of
action
potentials
of
individual
surface
fibres
in
response
to
external
electrical
stimulation
was
recorded.
In
a
brief
communication
Jenden
&
Reger
(1962)
have
reported
a
complete
failure
of
excitability
and
mechanical
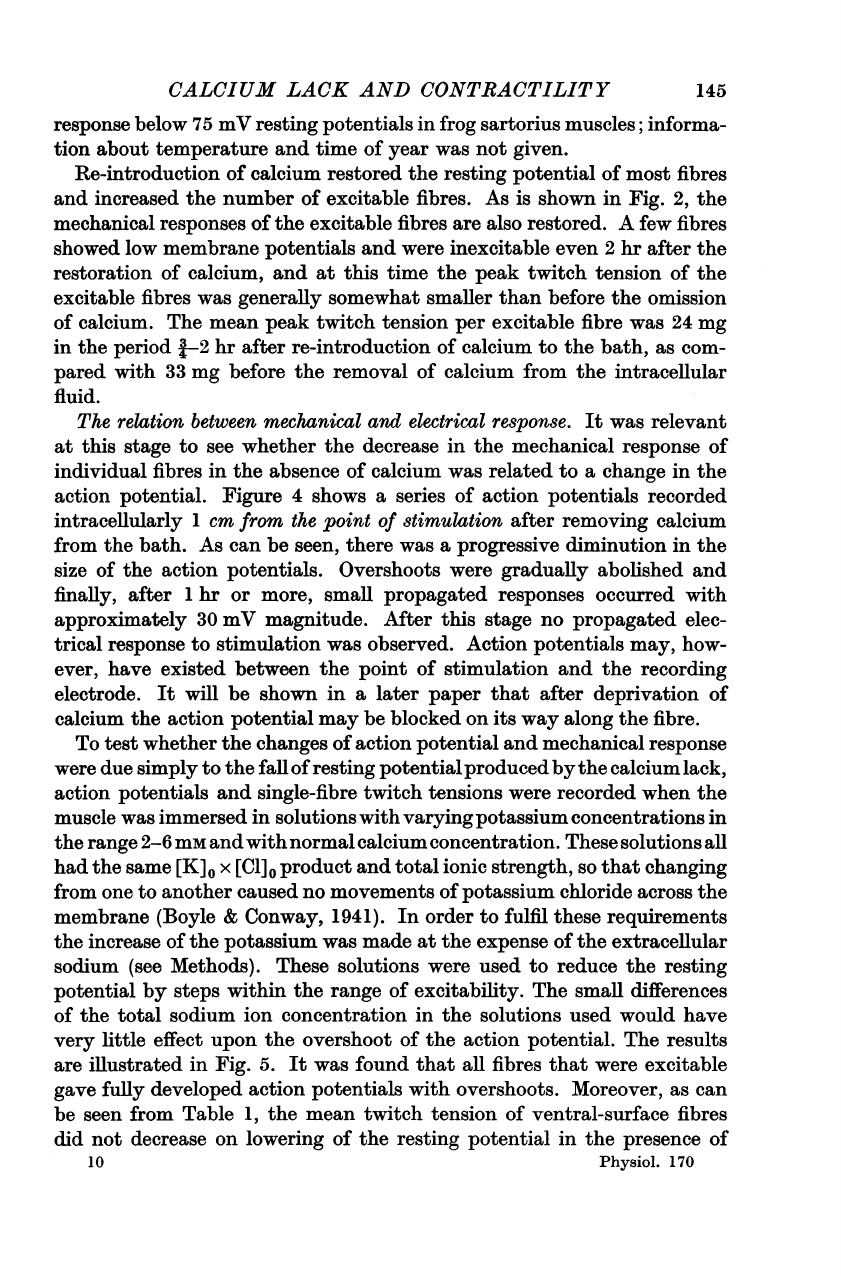
CALCIUM
LACK
AND
CONTRACTILITY
response
below
75
mV
resting
potentials
in
frog
sartorius
muscles;
informa-
tion
about
temperature
and
time
of
year
was
not
given.
Re-introduction
of
calcium
restored
the
resting
potential
of
most
fibres
and
increased
the
number
of
excitable
fibres.
As
is
shown
in
Fig.
2,
the
mechanical
responses
of
the
excitable
fibres
are
also
restored.
A
few
fibres
showed
low
membrane
potentials
and
were
inexcitable
even
2
hr
after
the
restoration
of
calcium,
and
at
this
time
the
peak
twitch
tension
of
the
excitable
fibres
was
generally
somewhat
smaller
than
before
the
omission
of
calcium.
The
mean
peak
twitch
tension
per
excitable
fibre
was
24
mg
in
the
period
1-2
hr
after
re-introduction
of
calcium
to
the
bath,
as
com-
pared
with
33
mg
before
the
removal
of
calcium
from
the
intracellular
fluid.
The
relation
between
mechanical
and
electrical
response.
It
was
relevant
at
this
stage
to
see
whether
the
decrease
in
the
mechanical
response
of
individual
fibres
in
the
absence
of
calcium
was
related
to
a
change
in
the
action
potential.
Figure
4
shows
a
series
of
action
potentials
recorded
intracellularly
1
cm
from
the
point
of
stimulation
after
removing
calcium
from
the
bath.
As
can
be
seen,
there
was
a
progressive
diminution
in
the
size
of
the
action
potentials.
Overshoots
were
gradually
abolished
and
finally,
after
1
hr
or
more,
small
propagated
responses
occurred
with
approximately
30
mV
magnitude.
After
this
stage
no
propagated
elec-
trical
response
to
stimulation
was
observed.
Action
potentials
may,
how-
ever,
have
existed
between
the
point
of
stimulation
and
the
recording
electrode.
It
will
be
shown
in
a
later
paper
that
after
deprivation
of
calcium
the
action
potential
may
be
blocked
on
its
way
along
the
fibre.
To
test
whether
the
changes
of
action
potential
and
mechanical
response
were
due
simply
to
the
fall
of
resting
potentialproduced
bythe
calcium
lack,
action
potentials
and
single-fibre
twitch
tensions
were
recorded
when
the
muscle
was
immersed
in
solutions
with
varyingpotassium
concentrations
in
the
range
2-6
mm
and
with
normal
calcium
concentration.
These
solutions
all
had
the
same
[K]0
x
[Cl]0
product
and
total
ionic
strength,
so
that
changing
from
one
to
another
caused
no
movements
of
potassium
chloride
across
the
membrane
(Boyle
&
Conway,
1941).
In
order
to
fulfil
these
requirements
the
increase
of
the
potassium
was
made
at
the
expense
of
the
extracellular
sodium
(see
Methods).
These
solutions
were
used
to
reduce
the
resting
potential
by
steps
within
the
range
of
excitability.
The
small
differences
of
the
total
sodium
ion
concentration
in
the
solutions
used
would
have
very
little
effect
upon
the
overshoot
of
the
action
potential.
The
results
are
illustrated
in
Fig.
5.
It
was
found
that
all
fibres
that
were
excitable
gave
fully
developed
action
potentials
with
overshoots.
Moreover,
as
can
be
seen
from
Table
1,
the
mean
twitch
tension
of
ventral-surface
fibres
did
not
decrease
on
lowering
of
the
resting
potential
in
the
presence
of
10
Physiol.
170
145
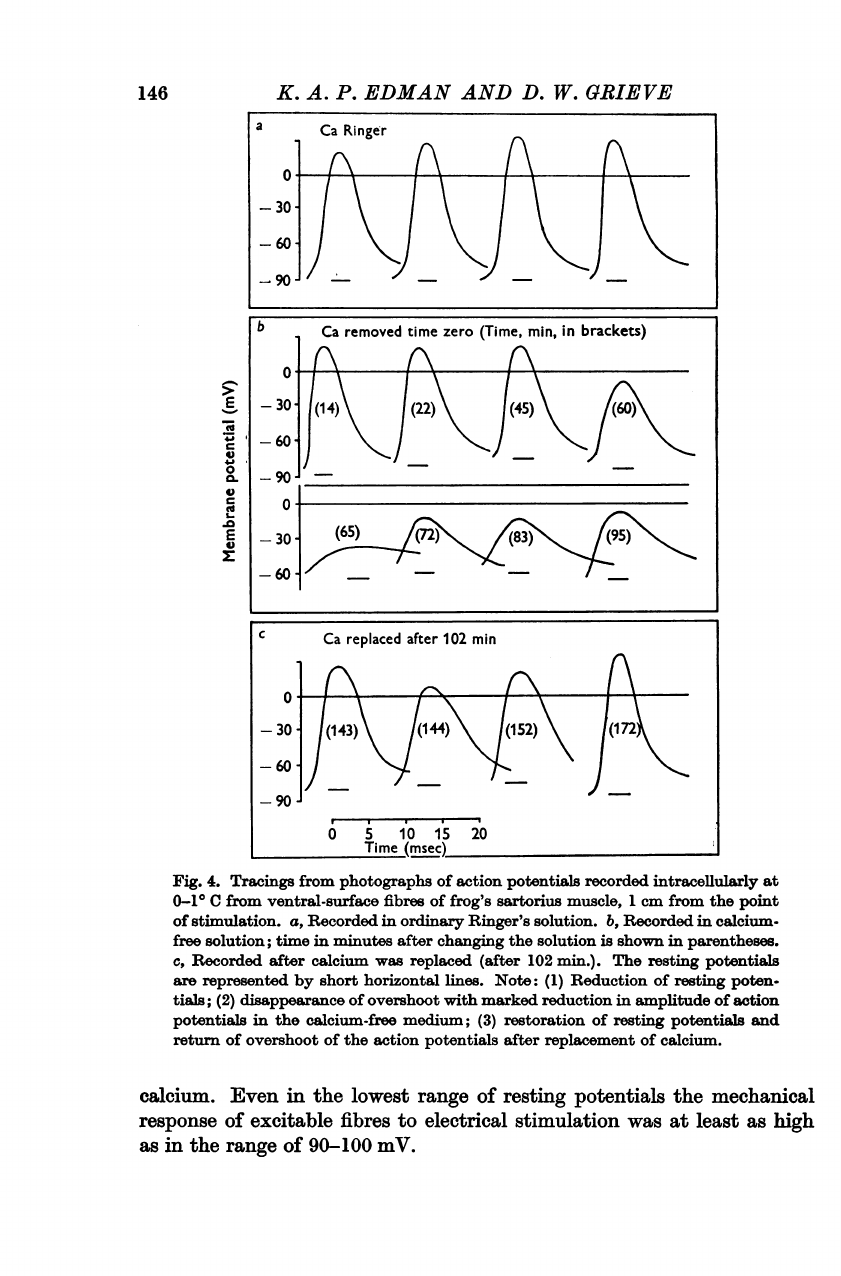
146
-.
0
0.
Cd
.0
E
a)
K.
A.
P.
EDMAN
AND
D.
W.
GRIEVE
a
Ca
Ringer
-
30
-A
C
Ca
replaced
after
102
min
-
30-
(143)
144)
(52)
(172
0
S
10
15
20
Time
(msec)
Fig.
4.
Tracings
from
photographs
of
action
potentials
recorded
intracellularly
at
0-1°
C
from
ventral-surface
fibres
of
frog's
sartorius
muscle,
1
cm
from
the
point
of
stimulation.
a,
Recorded
in
ordinary
Ringer's
solution.
b,
Recorded
in
calcium-
free
solution;
time
in
minutes
after
changing
the
solution
is
shown
in
parentheses.
c,
Recorded
after
calcium
was
replaced
(after
102
min.).
The
resting
potentials
are
represented
by
short
horizontal
lines.
Note:
(1)
Reduction
of
resting
poten-
tials;
(2)
disappearance
of
overshoot
with
marked
reduction
in
amplitude
of
action
potentials
in
the
calcium-free
medium;
(3)
restoration
of
resting
potentials
and
return
of
overshoot
of
the
action
potentials
after
replacement
of
calcium.
calcium.
Even
in
the
lowest
range
of
resting
potentials
the
mechanical
response
of
excitable
fibres
to
electrical
stimulation
was
at
least
as
high
as
in
the
range
of
90-100
mV.
b
Ca
removed
time
zero
(Time,
min,
in
brackets)
-30-
(14)
(22)
(45)
(60
-
60-
_90
-30-
(65)
((
-
60-
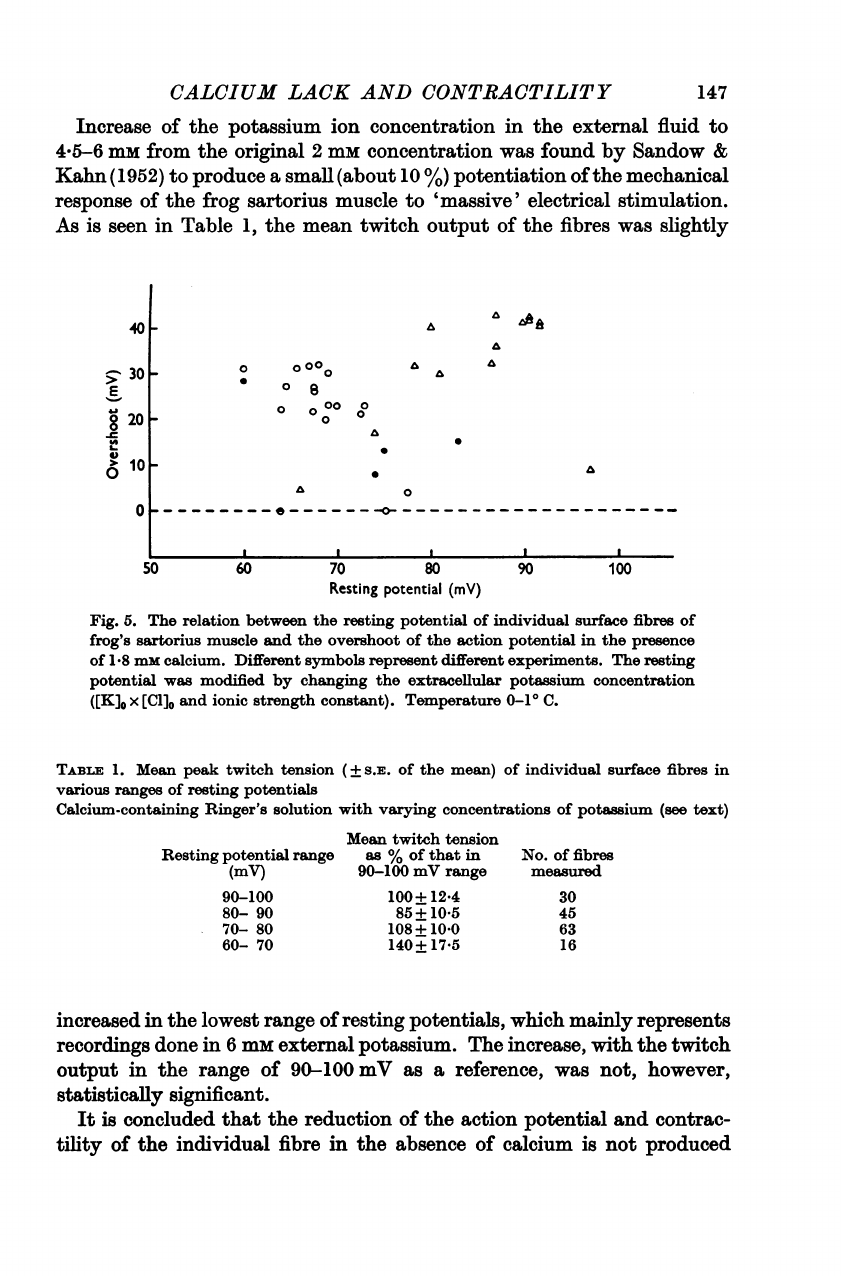
CALCIUM
LACK
AND
CONTRACTILITY
147
Increase
of
the
potassium
ion
concentration
in
the
external
fluid
to
45-6
mi
from
the
original
2
mm
concentration
was
found
by
Sandow
&
Kahn
(1952)
to
produce
a
small
(about
10
%)
potentiation
of
the
mechanical
response
of
the
frog
sartorius
muscle
to
'massive'
electrical
stimulation.
As
is
seen
in
Table
1,
the
mean
twitch
output
of
the
fibres
was
slightly
40
301-
201-
E
0
0
0
000
0
00
0
A
0
101-
0
0
A
a
a
a
a
0
.0-------
_
___________________
50
60
70
80
Resting
potential
(mV)
90
100
Fig.
5.
The
relation
between
the
resting
potential
of
individual
surface
fibres
of
frog's
sartorius
muscle
and
the
overshoot
of
the
action
potential
in
the
presence
of
1.8
mM
calcium.
Different
symbols
represent
different
experiments.
The
resting
potential
was
modified
by
changing
the
extracellular
potassium
concentration
([K]0
x
[Cl]0
and
ionic
strength
constant).
Temperature
0-1°
C.
TABLE
1.
Mean
peak
twitch
tension
(+
S.E.
of
the
mean)
of
individual
surface
fibres
in
various
ranges
of
resting
potentials
Calcium-containing
Ringer's
solution
with
varying
concentrations
of
potassium
(see
text)
Resting
potential
range
(mV)
90-100
80-
90
70-
80
60-
70
Mean
twitch
tension
as
%
of
that
in
90-100
mV
range
100+
12*4
85+
105
108
+
10*0
140+
17'5
increased
in
the
lowest
range
of
resting
potentials,
which
mainly
represents
recordings
done
in
6
mM
external
potassium.
The
increase,
with
the
twitch
output
in
the
range
of
90-100
mV
as
a
reference,
was
not,
however,
statistically
significant.
It
is
concluded
that
the
reduction
of
the
action
potential
and
contrac-
tility
of
the
individual
fibre
in
the
absence
of
calcium
is
not
produced
No.
of
fibres
measured
30
45
63
16
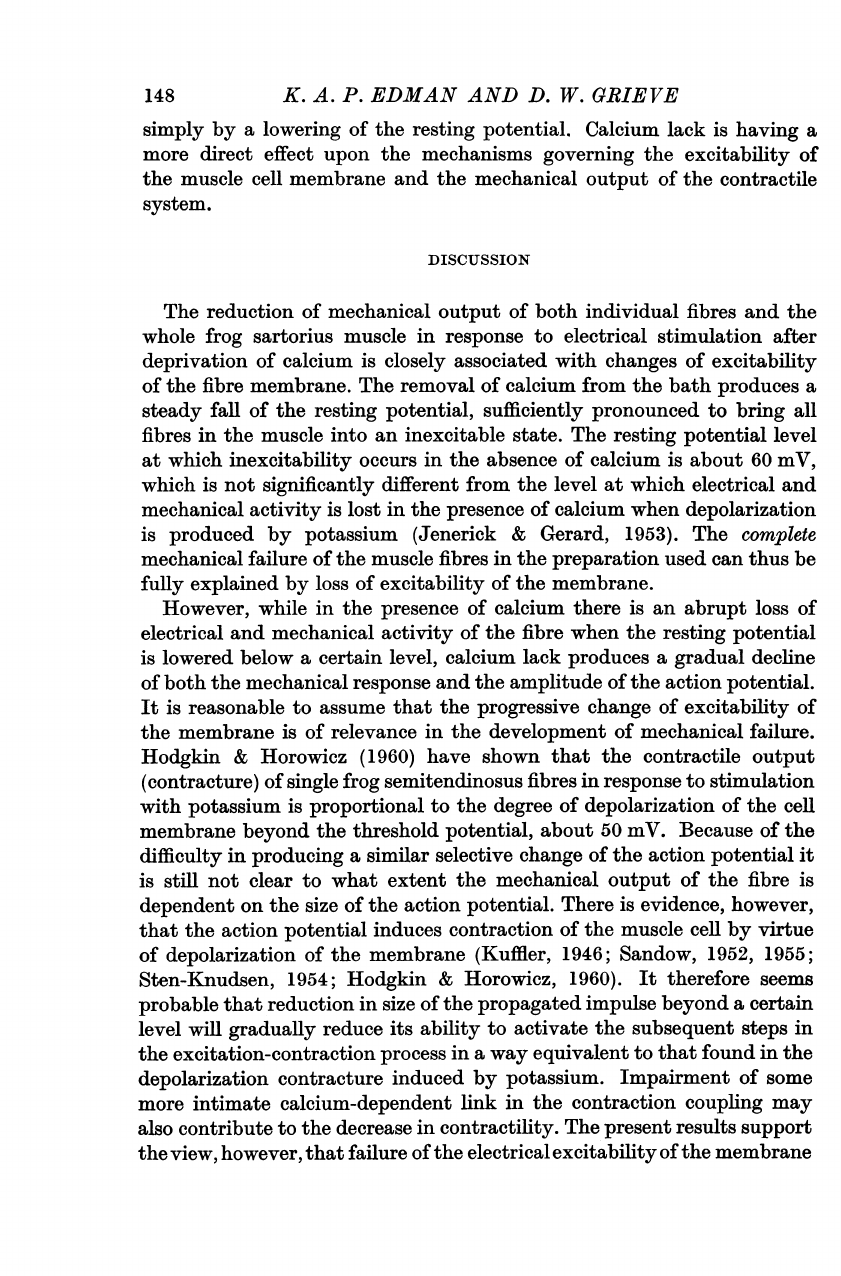
K.
A.
P.
EDMAN
AND
D.
W.
GRIEVE
simply
by
a
lowering
of
the
resting
potential.
Calcium
lack
is
having
a
more
direct
effect
upon
the
mechanisms
governing
the
excitability
of
the
muscle
cell
membrane
and
the
mechanical
output
of
the
contractile
system.
DISCUSSION
The
reduction
of
mechanical
output
of
both
individual
fibres
and
the
whole
frog
sartorius
muscle
in
response
to
electrical
stimulation
after
deprivation
of
calcium
is
closely
associated
with
changes
of
excitability
of
the
fibre
membrane.
The
removal
of
calcium
from
the
bath
produces
a
steady
fall
of
the
resting
potential,
sufficiently
pronounced
to
bring
all
fibres
in
the
muscle
into
an
inexcitable
state.
The
resting
potential
level
at
which
inexcitability
occurs
in
the
absence
of
calcium
is
about
60
mV,
which
is
not
significantly
different
from
the
level
at
which
electrical
and
mechanical
activity
is
lost
in
the
presence
of
calcium
when
depolarization
is
produced
by
potassium
(Jenerick
&
Gerard,
1953).
The
complete
mechanical
failure
of
the
muscle
fibres
in
the
preparation
used
can
thus
be
fully
explained
by
loss
of
excitability
of
the
membrane.
However,
while
in
the
presence
of
calcium
there
is
an
abrupt
loss
of
electrical
and
mechanical
activity
of
the
fibre
when
the
resting
potential
is
lowered
below
a
certain
level,
calcium
lack
produces
a
gradual
decline
of
both
the
mechanical
response
and
the
amplitude
of
the
action
potential.
It
is
reasonable
to
assume
that
the
progressive
change
of
excitability
of
the
membrane
is
of
relevance
in
the
development
of
mechanical
failure.
Hodgkin
&
Horowicz
(1960)
have
shown
that
the
contractile
output
(contracture)
of
single
frog
semitendinosus
fibres
in
response
to
stimulation
with
potassium
is
proportional
to
the
degree
of
depolarization
of
the
cell
membrane
beyond
the
threshold
potential,
about
50
mV.
Because
of
the
difficulty
in
producing
a
similar
selective
change
of
the
action
potential
it
is
still
not
clear
to
what
extent
the
mechanical
output
of
the
fibre
is
dependent
on
the
size
of
the
action
potential.
There
is
evidence,
however,
that
the
action
potential
induces
contraction
of
the
muscle
cell
by
virtue
of
depolarization
of
the
membrane
(Kuffler,
1946;
Sandow,
1952,
1955;
Sten-Knudsen,
1954;
Hodgkin
&
Horowicz,
1960).
It
therefore
seems
probable
that
reduction
in
size
of
the
propagated
impulse
beyond
a
certain
level
will
gradually
reduce
its
ability
to
activate
the
subsequent
steps
in
the
excitation-contraction
process
in
a
way
equivalent
to
that
found
in
the
depolarization
contracture
induced
by
potassium.
Impairment
of
some
more
intimate
calcium-dependent
link
in
the
contraction
coupling
may
also
contribute
to
the
decrease
in
contractility.
The
present
results
support
the
view,
however,
that
failure
of
the
electrical
excitability
of
the
membrane
148
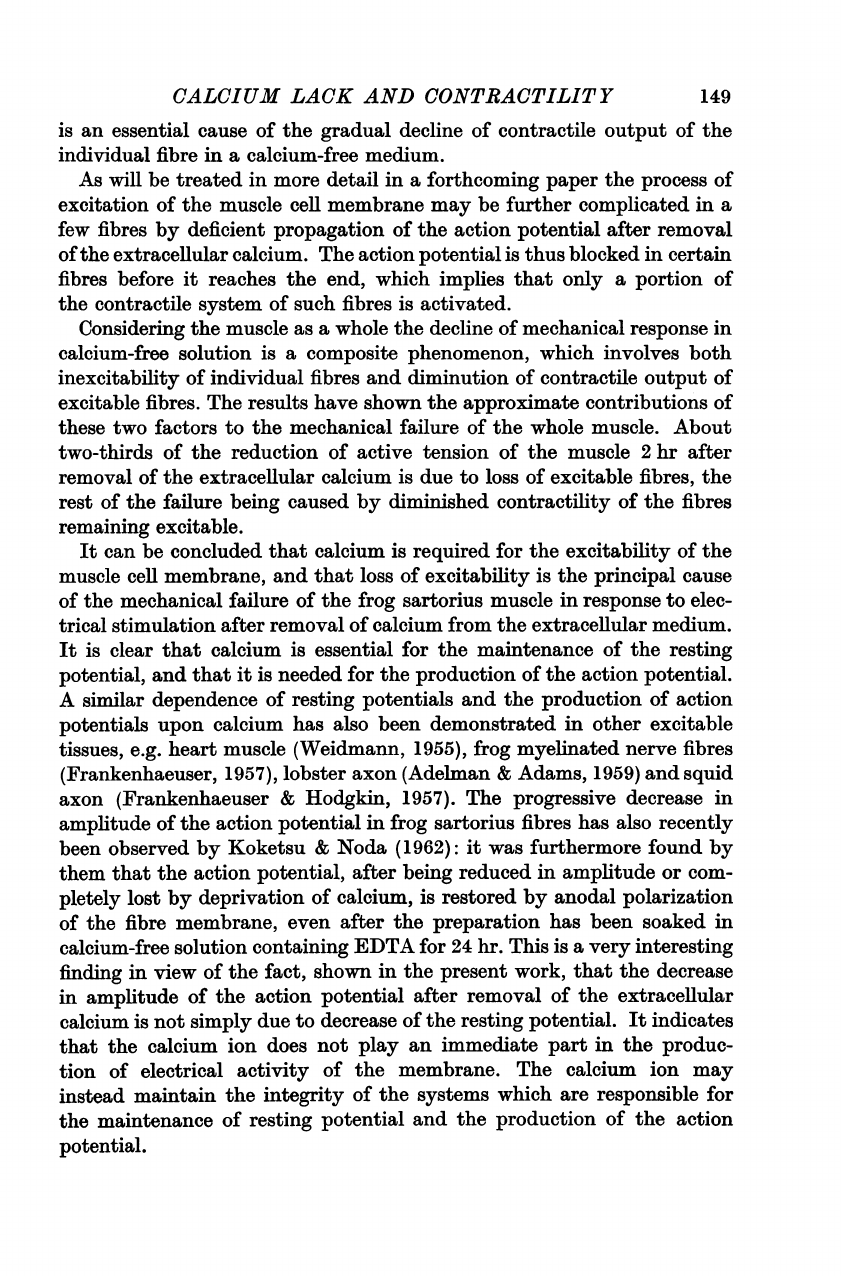
CALCIUM
LACK
AND
CONTRACTILITY
is
an
essential
cause
of
the
gradual
decline
of
contractile
output
of
the
individual
fibre
in
a
calcium-free
medium.
As
will
be
treated
in
more
detail
in
a
forthcoming
paper
the
process
of
excitation
of
the
muscle
cell
membrane
may
be
further
complicated
in
a
few
fibres
by
deficient
propagation
of
the
action
potential
after
removal
of
the
extracellular
calcium.
The
action
potential
is
thus
blocked
in
certain
fibres
before
it
reaches
the
end,
which
implies
that
only
a
portion
of
the
contractile
system
of
such
fibres
is
activated.
Considering
the
muscle
as
a
whole
the
decline
of
mechanical
response
in
calcium-free
solution
is
a
composite
phenomenon,
which
involves
both
inexcitability
of
individual
fibres
and
diminution
of
contractile
output
of
excitable
fibres.
The
results
have
shown
the
approximate
contributions
of
these
two
factors
to
the
mechanical
failure
of
the
whole
muscle.
About
two-thirds
of
the
reduction
of
active
tension
of
the
muscle
2
hr
after
removal
of
the
extracellular
calcium
is
due
to
loss
of
excitable
fibres,
the
rest
of
the
failure
being
caused
by
diminished
contractility
of
the
fibres
remaining
excitable.
It
can
be
concluded
that
calcium
is
required
for
the
excitability
of
the
muscle
cell
membrane,
and
that
loss
of
excitability
is
the
principal
cause
of
the
mechanical
failure
of
the
frog
sartorius
muscle
in
response
to
elec-
trical
stimulation
after
removal
of
calcium
from
the
extracellular
medium.
It
is
clear
that
calcium
is
essential
for
the
maintenance
of
the
resting
potential,
and
that
it
is
needed
for
the
production
of
the
action
potential.
A
similar
dependence
of
resting
potentials
and
the
production
of
action
potentials
upon
calcium
has
also
been
demonstrated
in
other
excitable
tissues,
e.g.
heart
muscle
(Weidmann,
1955),
frog
myelinated
nerve
fibres
(Frankenhaeuser,
1957),
lobster
axon
(Adelman
&
Adams,
1959)
and
squid
axon
(Frankenhaeuser
&
Hodgkin,
1957).
The
progressive
decrease
in
amplitude
of
the
action
potential
in
frog
sartorius
fibres
has
also
recently
been
observed
by
Koketsu
&
Noda
(1962):
it
was
furthermore
found
by
them
that
the
action
potential,
after
being
reduced
in
amplitude
or
com-
pletely
lost
by
deprivation
of
calcium,
is
restored
by
anodal
polarization
of
the
fibre
membrane,
even
after
the
preparation
has
been
soaked
in
calcium-free
solution
containing
EDTA
for
24
hr.
This
is
a
very
interesting
finding
in
view
of
the
fact,
shown
in
the
present
work,
that
the
decrease
in
amplitude
of
the
action
potential
after
removal
of
the
extracellular
calcium
is
not
simply
due
to
decrease
of
the
resting
potential.
It
indicates
that
the
calcium
ion
does
not
play
an
immediate
part
in
the
produc-
tion
of
electrical
activity
of
the
membrane.
The
calcium
ion
may
instead
maintain
the
integrity
of
the
systems
which
are
responsible
for
the
maintenance
of
resting
potential
and
the
production
of
the
action
potential.
149

K.
A.
P.
EDMAN
AND
D.
W.
GRIEVE
SUMMARY
1.
The
effects
of
calcium
lack
on
the
mechanical
output
of
whole
sar-
torius
muscle
and
of
individual
surface
fibres
of
the
frog
have
been
correlated
with
changes
of
the
resting
potentials
and
the
electrical
activity
of
the
individual
fibres.
2.
Removal
of
the
extracellular
calcium
produced
a
progressive
decline
of
the
resting
potentials
of
individual
fibres
to
equilibrium
values
of
about
40
mV.
3.
The
twitch
response
was
lost
after
the
resting
potential
had
been
reduced
to
about
60
mV,
i.e.
not
markedly
different
from
the
level
at
which
electrical
inexcitability
occurs
in
the
presence
of
calcium.
4.
Instead
of
an
all-or-nothing
failure
of
mechanical
and
electrical
activity,
as
occurs
in
the
presence
of
calcium,
there
is
a
gradual
decline
of
both
the
amplitude
of
the
action
potential
and
the
height
of
the
twitch
response
of
the
individual
muscle
cells
in
the
absence
of
calcium,
in
parallel
with
the
lowering
of
the
resting
potential.
After
2
hr
in
calcium-free
Ringer's
solution
55
%
of
the
fibres
were
inexcitable
and
the
mean
peak
twitch
tension
per
excitable
fibre
had
been
lowered
to
half
the
original
value
in
the
calcium-containiing
medium.
Overshoots
of
the
action
poten-
tials
were
gradually
abolished,
and
after
1
hr
or
more,
before
the
fibres
became
inexcitable,
propagated
impulses
of
approximately
30
mV
ampli-
tude
were
recorded.
5.
The
complete
loss
of
mechanical
output
of
individual
fibres
in
response
to
electrical
stimulation
in
the
absence
of
extracellular
calcium
can
be
fully
explained
by
inexcitability
of
the
cell
membrane
caused
by
reduction
of
the
resting
potential.
The
progressive
decline
of
the
twitch
response
of
the
single
fibre
which
precedes
the
complete
mechanical
failure
may
to
a
great
extent
be
accounted
for
by
deficient
activation
of
the
con-
tractile
system
by
the
diminished
action
potential.
REFERENCES
ADELMAN,
W.
J.
&
ADAmS,
J.
(1959).
Effects
of
calcium
lack
on
action
potential
of
motor
axons
of
the
lobster
limb.
J.
gen.
Phy8iol.
42,
655-664.
BIacm,
C.
P.
(1961
a).
Calcium
movements
in
muscle.
Circulation,
24,
518-522.
BuNcH,
C.
P.
(1961
b).
Calcium
movements
in
striated
muscle
during
contraction
and
contracture.
In
Biophys1ias
of
Phy8iological
and
Pharmacological
Action,
ed.
Shanes,
A.
M.,
pp.
281-292.
Washington:
American
Association
for
the
Advancement
of
Science,
Publication
No.
69.
BIrcm,
C.
P.
&
SHANEs,
A.
M.
(1959).
Calcium
influx
in
skeletal
muscle
at
rest,
during
activity
and
during
potassium
contracture.
J.
gen.
Physiol.
42,
803-815.
BoYLE,
P.
J.
&
CONWAY,
E.
J.
(1941).
Potassium
accumulation
in
muscle
and
associated
changes.
J.
Phy8iol.
100,
1-63.
150
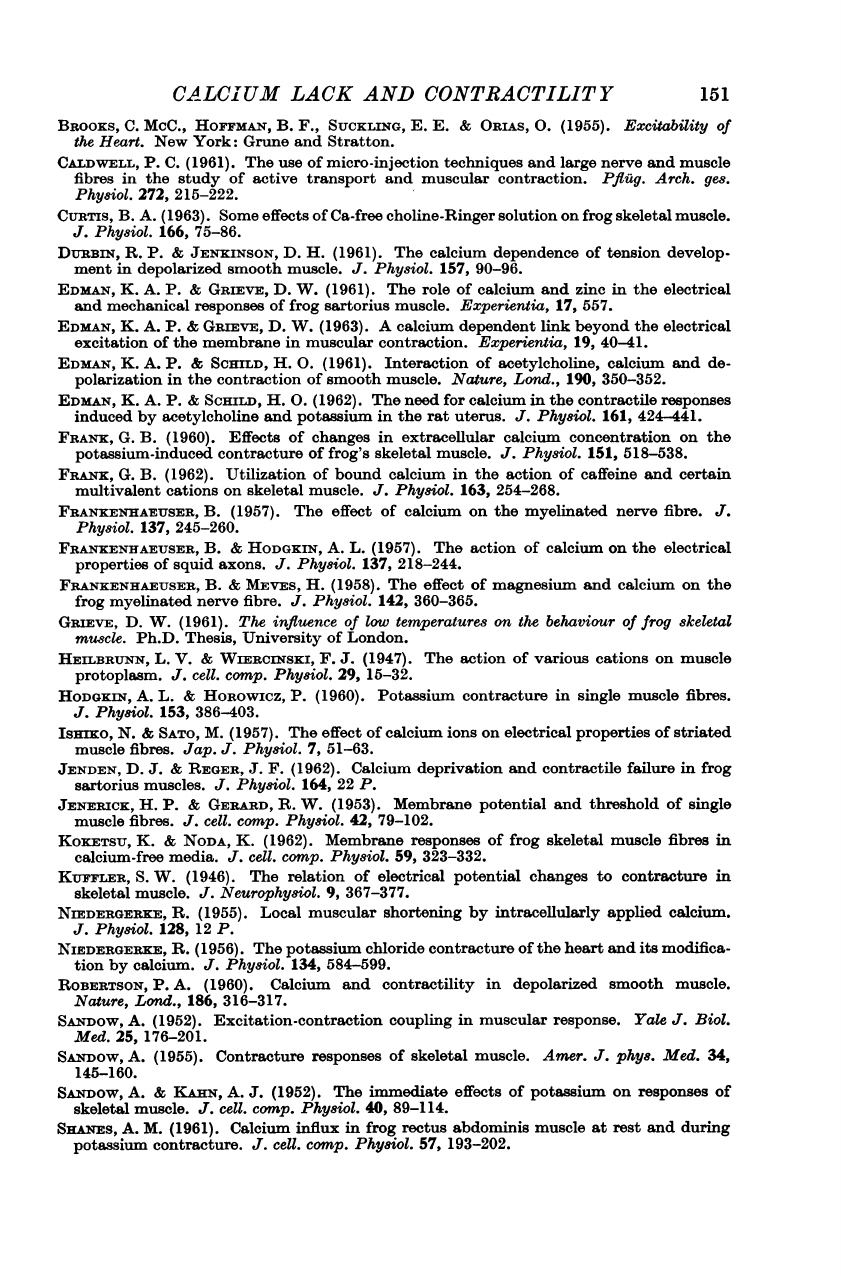
CALCIUM
LACK
AND
CONTRACTILITY
151
BROOKS,
C.
MCC.,
HOFFMAN,
B.
F.,
SUCKLING,
E.
E.
&
ORiAs,
0.
(1955).
Excitability
of
the
Heart.
New
York:
Grune
and
Stratton.
CATDWELL,
P.
C.
(1961).
The
use
of
micro-injection
techniques
and
large
nerve
and
muscle
fibres
in
the
study
of
active
transport
and
muscular
contraction.
Pflug.
Arch.
ges.
Physiol.
272,
215-222.
CuRTis,
B.
A.
(1963).
Some
effects
of
Ca-free
choline-Ringer
solution
on
frog
skeletal
muscle.
J.
Physiol.
166,
75-86.
DuRBIN,
R.
P.
&
JENsNSON,
D.
H.
(1961).
The
calcium
dependence
of
tension
develop-
ment
in
depolarized
smooth
muscle.
J.
Physiol.
157,
90-96.
EDMAN,
K.
A.
P.
&
GRIEVE,
D.
W.
(1961).
The
role
of
calcium
and
zinc
in
the
electrical
and
mechanical
responses
of
frog
sartorius
muscle.
Experientia,
17,
557.
EDmAN,
K.
A.
P.
&
GRmvE,
D.
W.
(1963).
A
calcium
dependent
link
beyond
the
electrical
excitation
of
the
membrane
in
muscular
contraction.
Experientia,
19,
40-41.
EDMAN,
K.
A.
P.
&
ScEHILD,
H.
0.
(1961).
Interaction
of
acetylcholine,
calcium
and
de-
polarization
in
the
contraction
of
smooth
muscle.
Nature,
Lond.,
190,
350-352.
EDMAN,
K.
A.
P.
&
Scmw,
H.
0.
(1962).
The
need
for
calcium
in
the
contractile
responses
induced
by
acetylcholine
and
potassium
in
the
rat
uterus.
J.
Physiol.
161,
424-441.
FRANK,
G.
B.
(1960).
Effects
of
changes
in
extraceilular
calcium
concentration
on
the
potassium-induced
contracture
of
frog's
skeletal
muscle.
J.
Phy8iol.
151,
518-538.
FRANx,
G.
B.
(1962).
Utilization
of
bound
calcium
in
the
action
of
caffeine
and
certain
multivalent
cations
on
skeletal
muscle.
J.
Physiol.
163,
254-268.
FRANKENHAEUSER,
B.
(1957).
The
effect
of
calcium
on
the
myelinated
nerve
fibre.
J.
Physiol.
137,
245-260.
FRANKENiAEUSER,
B.
&
HODGKIN,
A.
L.
(1957).
The
action
of
calcium
on
the
electrical
properties
of
squid
axons.
J.
Physiol.
137,
218-244.
FRANKENHAETusER,
B.
&
MxvEs,
H.
(1958).
The
effect
of
magnesium
and
calcium
on
the
frog
myelinated
nerve
fibre.
J.
Physiol.
142,
360-365.
GRIEVE,
D.
W.
(1961).
The
influence
of
low
temperatures
on
the
behaviour
of
frog
skeletal
muscle.
Ph.D.
Thesis,
University
of
London.
HEILBRuNN,
L.
V.
&
WIERcINsKI,
F.
J.
(1947).
The
action
of
various
cations
on
muscle
protoplasm.
J.
cell.
comp.
Physiol.
29,
15-32.
HODGKIN,
A.
L.
&
HOROWICZ,
P.
(1960).
Potassium
contracture
in
single
muscle
fibres.
J.
Physiol.
153,
386-403.
IsT
KO,
N.
&
SATO,
M.
(1957).
The
effect
of
calcium
ions
on
electrical
properties
of
striated
muscle
fibres.
Jap.
J.
Physiol.
7,
51-63.
JENDEN,
D.
J.
&
REGER,
J.
F.
(1962).
Calcium
deprivation
and
contractile
failure
in
frog
sartorius
muscles.
J.
Physiol.
164,
22
P.
JENERICK,
H.
P.
&
GERARD,
R.
W.
(1953).
Membrane
potential
and
threshold
of
single
muscle
fibres.
J.
cell.
comp.
Physiol.
42,
79-102.
KOKETSU,
K.
&
NODA,
K.
(1962).
Membrane
responses
of
frog
skeletal
muscle
fibres
in
calcium-free
media.
J.
cell.
comp.
Physiol.
59,
323-332.
KuFFLER,
S.
W.
(1946).
The
relation
of
electrical
potential
changes
to
contracture
in
skeletal
muscle.
J.
Neurophysiol.
9,
367-377.
NIEDERGER,
R.
(1955).
Local
muscular
shortening
by
intracellularly
applied
calcium.
J.
Physiol.
128,
12
P.
NIEDERGER,
R.
(1956).
The
potassium
chloride
contracture
of
the
heart
and
its
modifica-
tion
by
calcium.
J.
Physiol.
134,
584-599.
ROBERTSON,
P.
A.
(1960).
Calcium
and
contractility
in
depolarized
smooth
muscle.
Nature,
Lond.,
186,
316-317.
SANDow,
A.
(1952).
Excitation-contraction
coupling
in
muscular
response.
Yale
J.
Biol.
Med.
25,
176-201.
SANDow,
A.
(1955).
Contracture
responses
of
skeletal
muscle.
Amer.
J.
phys.
Med.
34,
145-160.
SANDoW,
A.
&
KAHN,
A.
J.
(1952).
The
immediate
effects
of
potassium
on
responses
of
skeletal
muscle.
J.
cell.
comp.
Physiol.
40,
89-114.
SHAwEs,
A.
M.
(1961).
Calcium
influx
in
frog
rectus
abdominis
muscle
at
rest
and
during
potassium
contracture.
J.
cell.
comp.
Physiol.
57,
193-202.
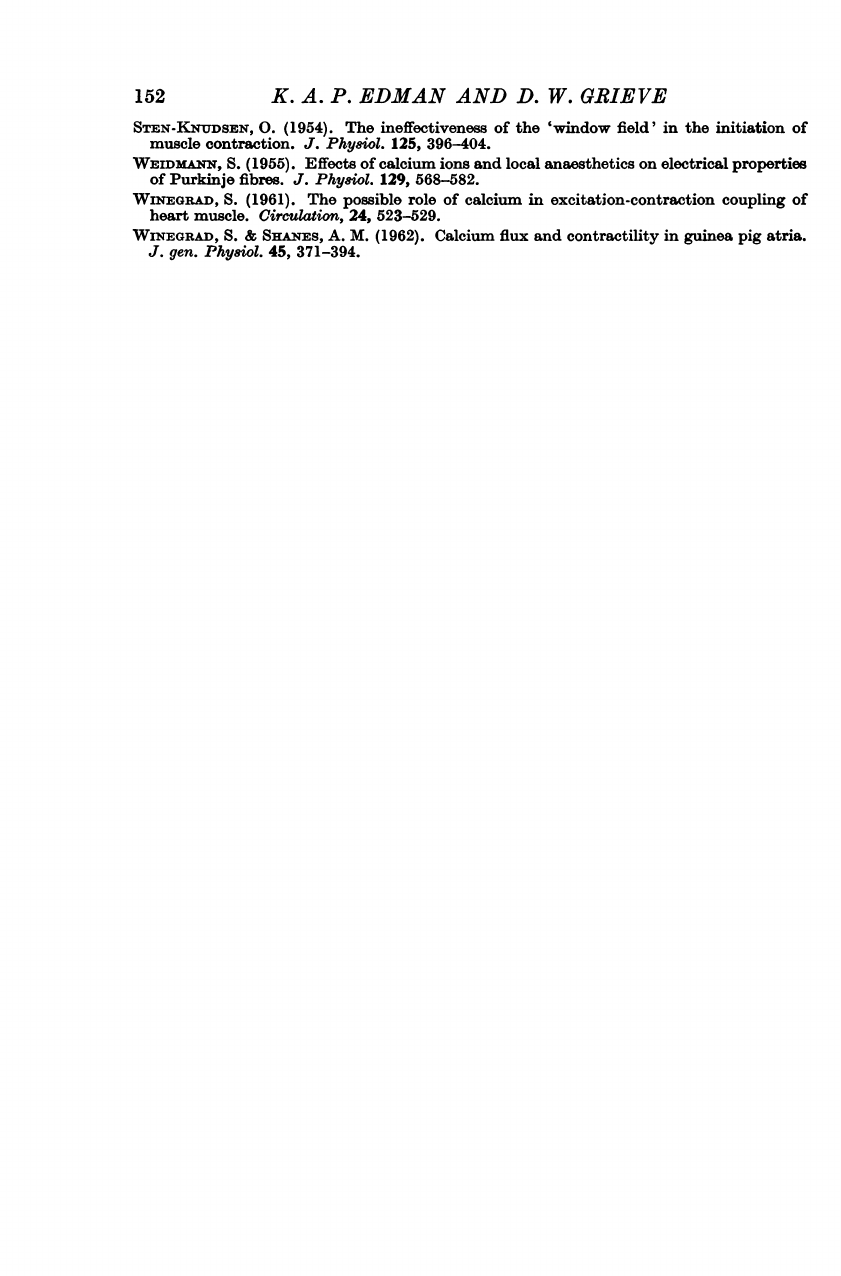
152
K.
A.
P.
EDMAN
AND
D.
W.
GRIEVE
STEN-KNUDsEN,
0.
(1954).
The
ineffectiveness
of
the
'window
field'
in
the
initiation
of
muscle
contraction.
J.
Phy8iol.
125,
396-404.
WEIDmANN,
S.
(1955).
Effects
of
calcium
ions
and
local
anaesthetics
on
electrical
properties
of
Purkinje
fibres.
J.
Phy8iol.
129,
568-582.
WnMGaRAD,
S.
(1961).
The
possible
role
of
calcium
in
excitation-contraction
coupling
of
heart
muscle.
Circulation,
24,
523-529.
WINEGRAD,
S.
&
SHANNEs,
A.
M.
(1962).
Calcium
flux
and
contractility
in
guinea
pig
atria.
J.
gen.
Physiot.
45,
371-394.