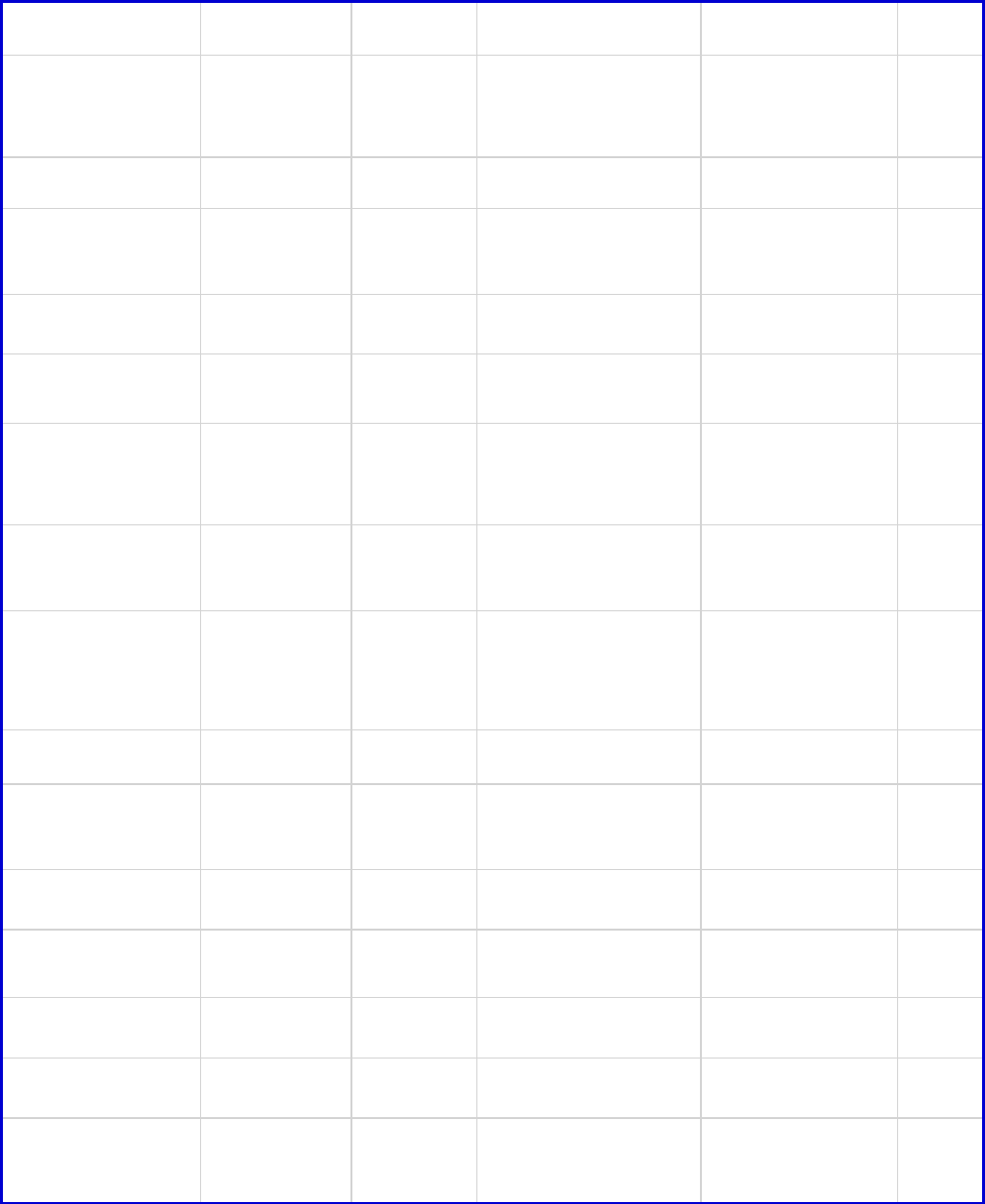
16
Table 1: Descriptions for indicators used in the IWSSRR/EA Vulnerability Tool analysis.
Indicator Short Name
Indicator Name
Large Values = High
Vulnerability
Indicator Description
Data Sources
Last
8 AT RISK FRESHWATER PLANT
% of freshwater plant
communities at risk
Yes
% of wetlands & riparian plant
communities that are at risk of
extinction, based on remaining number
& condition, remaining acreage, threat
severity, etc.
NatureServe - Explorer (customized
dataset). Data were obtained from
Jason McNees at NatureServe,
1101 Wilson Blvd., 15th Floor
Arlington, VA 22201 via email on
July 31, 2009
Feb-2016
65L MEAN ANNUAL RUNOFF
Mean annual runoff
(local)
No
Mean runoff: average annual runoff,
excluding upstream freshwater inputs
Data calculated from interagency
CMIP5 GCM - BCSD - VIC dataset
Sep-2014
95 DROUGHT SEVERITY
Drought Severity Index
Yes
Greatest precipitation deficit: The most
negative value calculated by
subtracting potential
evapotranspiration from precipitation
over any 1-, 3-, 6-, or 12-month period.
Data calculated from interagency
CMIP5 GCM - BCSD - VIC dataset
(2014)
Jul-2015
156 SEDIMENT
load due to change in
Yes
The ratio of the change in the sediment
load in the future to the present load.
CDM
Feb-2016
175L ANNUAL COV
Annual CV of
unregulated runoff
(local)
Yes
Long-term variability in hydrology:
ratio of the SD of annual runoff to the
annual runoff mean. Excludes upstream
freshwater inputs (local).
Data calculated from interagency
CMIP5 GCM - BCSD - VIC dataset
(2014)
Sep-2014
221C MONTHLY COV
Monthly CV of runoff
(cumulative)
Yes
Measure of short-term variability in the
region's hydrology: 75th percentile of
annual ratios of the SD of monthly
runoff to the mean of monthly runoff.
Includes upstream freshwater inputs
Data calculated from interagency
CMIP5 GCM - BCSD - VIC dataset
(2014)
Sep-2014
277 RUNOFF PRECIP
% change in runoff
divided by % change in
precipitation
Yes
Median of: deviation of runoff from
monthly mean times average monthly
runoff divided by deviation of
precipitation from monthly mean times
average monthly precipitation.
Data calculated from interagency
CMIP5 GCM - BCSD - VIC dataset
(2014) using method of
Sankarasubramanian & Vogel
Feb-2015
297 MACROINVERTEBRATE
Macroinvertebrate
index of biotic
condition
No
The sum (ranging from 0-100) of scores
for six metrics that characterize
macroinvertebrate assemblages:
taxonomic richness, taxonomic
composition, taxonomic diversity,
feeding groups, habits, pollution
tolerance.
USEPA - Wadeable Streams
Assesment (WSA) (Stream Water
Benthic Macroinvertebrate
Metrics)
Feb-2016
568C FLOOD MAGNIFICATION
Flood magnification
factor (cumulative)
Yes
Change in flood runoff: ratio of
indicator 571C (monthly runoff
exceeded 10% of the time, including
Data calculated from interagency
CMIP5 GCM - BCSD - VIC dataset
(2014)
Sep-2014
568L FLOOD MAGNIFICATION
Flood magnification
factor (local)
Yes
Change in flood runoff: Ratio of
indicator 571L (monthly runoff
exceeded 10% of the time, excluding
upstream freshwater inputs) to 571L in
Data calculated from interagency
CMIP5 GCM - BCSD - VIC dataset
(2014)
Sep-2014
570L 90PERC EXCEEDANCE
flow exceeded 90% of
No
Low runoff: monthly runoff that is
exceeded 90% of the time, excluding
upstream freshwater inputs (local).
Data calculated from interagency
CMIP5 GCM - BCSD - VIC dataset
(2014)
Sep-2014
571C 10PERC EXCEEDANCE
Flood flow (monthly
flow exceeded 10% of
time; cumulative)
Yes
Flood runoff: monthly runoff that is
exceeded 10% of the time, including
upstream freshwater inputs
Data calculated from interagency
CMIP5 GCM - BCSD - VIC dataset
(2014)
Sep-2014
571L 10PERC EXCEEDANCE
flow exceeded 10% of
Yes
Flood runoff: monthly runoff that is
exceeded 10% of the time, excluding
upstream freshwater inputs (local).
Data calculated from interagency
CMIP5 GCM - BCSD - VIC dataset
(2014)
Sep-2014
590 URBAN 500YRFLOODPLAIN
AREA
within 500-year
Yes
Acres of urban area within the 500-year
floodplain.
(1)
FEMA - 500 year Flood Zones
(2)
EPA - Integrated Climate & L&
Use Scenarios (ICLUS)
Jan-2011
700C LOW FLOW REDUCTION
Low flow reduction
factor (cumulative)
No
Change in low runoff: ratio of indicator
570C (monthly runoff exceeded 90% of
the time, including upstream
freshwater inputs) to 570C in base
Data calculated from interagency
CMIP5 GCM - BCSD - VIC dataset
(2014)
Sep-2014