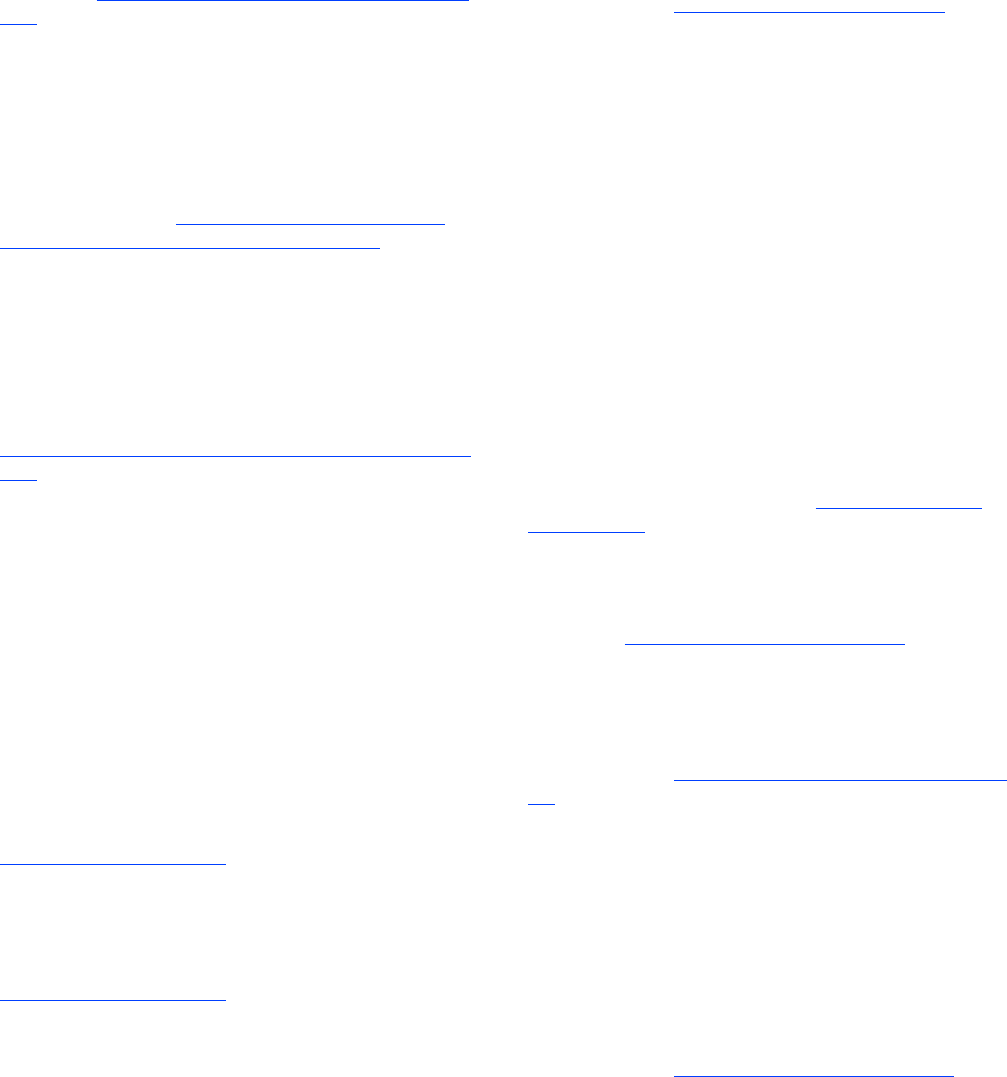
72 VOC Blank Data and Study Reporting Levels, California GAMA PBP, 2004–2010
Childress, C.J.O., Foreman, W.T., Connor, B.F., and Maloney,
T.J., 1999, New reporting procedures based on long-term
method detection levels and some considerations for
interpretations of water-quality data provided by the U.S.
Geological Survey National Water Quality Laboratory: U.S.
Geological Survey Open-File Report 99-193, 19 p. (Also
available at http://water.usgs.gov/owq/OFR_99-193/index.
html.)
Chin, M., and Davis, D.D., 1993, Global sources and
sinks of OCS and CS
2
and their distributions: Global
Biogeochemical Cycles, v. 7, p. 321–337.
City of Sacramento, 2010, 2010 Water quality report—A
consumer condence report for the citizens of Sacramento:
City of Sacramento Department of Utilities, accessed
December 2, 2011, at http://www.cityofsacramento.org/
utilities/water/documents/CCR_LINO_web.pdf.
Connor, B.F., Rose, D.L., Noriega, M.C., Murtaugh, L.K.,
and Abney, S.R., 1998, Methods of analysis by the U.S.
Geological Survey National Water Quality Laboratory—
Determination of 86 volatile organic compounds in water
by gas chromatography/mass spectrometry, including
detections less than reporting limits: U.S. Geological
Survey Open-File Report 97-829, 78 p. (Also available at
http://nwql.usgs.gov/Public/pubs/OFR97-829/OFR97-829.
html.)
Daisey, J.M., Hodgson, A.T., Fisk, W.J., Mendell, M.J.,
and Ten Brinke, J., 1994, Volatile organic compounds in
twelve California ofce buildings—Classes, concentrations
and sources: Atmospheric Environment, v. 28, no. 22,
p. 3557–3562.
Devai, I., and DeLaune, R.D., 1995, Formation of volatile
sulfur compounds in salt marsh sediment as inuenced by
soil redox condition: Organic Geochemistry, v. 23, no. 4,
p. 283–287.
Ferrari, M.J., Fram, M.S., and Belitz, Kenneth, 2008, Ground-
water quality in the Central Sierra study unit, California,
2006—Results from the California GAMA Program: U.S.
Geological Survey Data Series 335, 60 p. (Also available at
http://pubs.usgs.gov/ds/335/.)
Fram, M.S., Munday, C.M., and Belitz, Kenneth, 2009,
Groundwater quality data for the Tahoe-Martis study unit,
2007—Results from the California GAMA Program: U.S.
Geological Survey Data Series 432, 88 p. (Also available at
http://pubs.usgs.gov/ds/432/.)
Hahn, G.J., and Meeker, W.Q., 1991, Statistical intervals—A
guide to practitioners: New York, John Wiley and Sons,
392 p.
Helsel, D.R., 2005, Nondetects and data analysis—Statistics
for censored environmental data: New York, John Wiley
and Sons, 268 p.
Helsel, D.R., and Hirsch, R.M., 2002, Statistical methods in
water resources: U.S. Geological Survey Techniques of
Water-Resources Investigations, book 4, chap. A3, 510 p.
(Also available at http://pubs.usgs.gov/twri/twri4a3/.)
Horton, T.R., 1983, Methods and results of EPA’s study
of radon in drinking water: U.S. Environmental
Protection Agency Ofce of Radiation Programs, Eastern
Environmental Radiation Facility, Montomery, Ala., EPA-
520/5-83-027, 25 p.
J&W Scientic, 1998, Catalog, technical reference,
and cookbook: Folsom, California, J&W Scientic
Incorporated, 420 p.
Landon, M.K., and Belitz, Kenneth, 2012, Geogenic
sources of benzene in aquifers used for public supply,
California: Environmental Science and Technology, v. 46,
p. 8689–8697.
Landon, M.K., Belitz, Kenneth, Jurgens, B.C., Kulongoski,
J.T., and Johnson, T.D., 2010, Status and understanding of
groundwater quality in the Central–Eastside San Joaquin
Basin, 2006—California GAMA Priority Basin Project:
U.S. Geological Survey Scientic Investigations Report
2009-5266, 97 p. (Also available at http://pubs.usgs.gov/
sir/2009/5266/.)
Maloney, T.J., ed., 2005, Quality management system, U.S.
Geological Survey National Water Quality Laboratory: U.S.
Geological Survey Open-File Report 2005-1263. (Also
available at http://pubs.usgs.gov/of/2005/1263/.)
Martin, J.D., Gilliom, R.J., and Schertz, T.L., 1999, Summary
and evaluation of pesticides in eld blanks collected for
the National Water-Quality Assessment Program, 1992–95:
U.S. Geological Survey Open-File Report 98-412, 102 p.
(Also available at http://pubs.usgs.gov/of/1998/0412/report.
pdf.)
Monod, Anne, Sive, B.C., Avino, Pasquale, Chen, Tai, Blake,
D.R., and Rowland, F.S., 2001, Monoaromatic compounds
in ambient air of various cities—A focus on correlations
between the xylenes and ethylbenzene: Atmospheric
Environment, v. 35, p. 135–149.
Moran, M.J., Zogorski, J.S., and Rowe, B.L., 2006, Approach
to an assessment of volatile organic compounds in the
nation’s ground water and drinking-water supply wells:
U.S. Geological Survey Open-File Report 2005-1452, 36 p.
(Also available at http://pubs.usgs.gov/of/2005/1452/.)