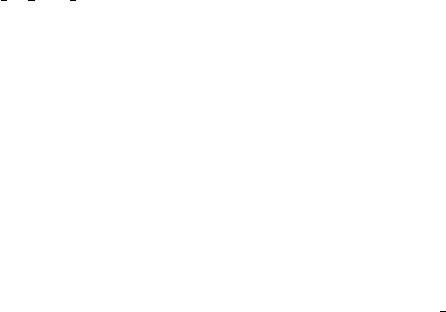
manuscript submitted to Geophysical Research Letters
Domeisen, D. I., Butler, A. H., Charlton-Perez, A. J., Ayarzag¨uena, B., Baldwin,303
M. P., Dunn-Sigouin, E., . . . Taguchi, M. (2019). The role of the strato-304
sphere in subseasonal to seasonal prediction Part II: Predictability arising from305
stratosphere - troposphere coupling. J. Geophys. Res., n/a(n/a). Retrieved306
from https://agupubs.onlinelibrary.wiley.com/doi/abs/10.1029/307
2019JD030923 doi: 10.1029/2019JD030923308
Gelaro, R., McCarty, W., Surez, M. J., Todling, R., Molod, A., Takacs, L., . . .309
Zhao, B. (2017). The Modern-Era Retrospective Analysis for Research310
and Applications, Version-2 (MERRA-2). J. Clim., 30 , 5419–5454. doi:311
doi:10.1175/JCLI-D-16-0758.1312
Global Modeling and Assimilation Office (GMAO). (2015). Merra-2313
inst3 3d asm nv: 3d, 3-hourly,instantaneous, model-level, assimilation, as-314
similated meteorological fields v5.12.4, greenbelt, md, usa, Goddard Earth Sci-315
ences Data and Information Services Center (GES DISC), accessed 1 novem-316
ber 2015. doi: 10.5067/WWQSXQ8IVFW8317
Hoskins, B. J., McIntyre, M. E., & Robertson, A. W. (1985). On the use and signifi-318
cance of isentropic potential vorticity maps. Q. J. R. Meteorol. Soc., 111 , 877–319
946.320
Huang, J., Hitchcock, P., Maycock, A. C., McKenna, C. M., & Tian, W. (2021,321
July). Northern hemisphere cold air outbreaks are more likely to be severe322
during weak polar vortex conditions. Comm. Earth Env., 2 (1), 147. Retrieved323
from https://doi.org/10.1038/s43247-021-00215-6324
Jiang, J. H. (2021). Polar vortex linked to atmospheric circulation at daily scale.325
Retrieved from https://eos.org/editor-highlights/polar-vortex-linked326
-to-atmospheric-circulation-at-daily-scale?utm campaign=ealert327
(EOS Editors’ Highlight)328
Juzbaˇsi´c, A., Kryjov, V. N., & Ahn, J. B. (2021, apr). On the anomalous devel-329
opment of the extremely intense positive Arctic oscillation of the 2019–2020330
winter. Env. Res. Lett., 16 (5), 055008. Retrieved from https://doi.org/331
10.1088/1748-9326/abe434 doi: 10.1088/1748-9326/abe434332
Kidston, J., Scaife, A. A., Hardiman, S. C., Mitchell, D. M., Butchart, N., Bald-333
win, M. P., & Gray, L. J. (2015). Stratospheric influence on tropospheric334
jet streams, storm tracks and surface weather. Nature Geoscience, 8 . doi:335
http://dx.doi.org/10.1038/ngeo2424336
King, A. D., Butler, A. H., Jucker, M., Earl, N. O., & Rudeva, I. (2019). Observed337
relationships between sudden stratospheric warmings and European climate338
extremes. J. Geophys. Res., 124 (24), 13943–13961. Retrieved from https://339
agupubs.onlinelibrary.wiley.com/doi/abs/10.1029/2019JD030480 doi:340
https://doi.org/10.1029/2019JD030480341
K¨om¨u¸sc¨u, A.
¨
U., & O˘guz, K. (2021, August). Analysis of cold anomalies observed342
over Turkey during the 2018/2019 winter in relation to polar vortex and other343
atmospheric patterns. Meteorol. Atmos. Phys., 133 (4), 1327–1354. Retrieved344
from https://doi.org/10.1007/s00703-021-00806-0345
Kretschmer, M., Cohen, J., Mattias, V., Runge, J., & Coumou, D. (2018). The dif-346
ferent stratospheric influence on cold-extremes in Eurasia and North America.347
npj Clim Atmos Sci, 1 . doi: 10.1038/s41612-018-0054-4348
Lawrence, Z. D., & Manney, G. L. (2018). Characterizing stratospheric polar349
vortex variability with computer vision techniques. Journal of Geophysical Re-350
search: Atmospheres, 123 (3), 1510–1535. Retrieved from http://dx.doi.org/351
10.1002/2017JD027556 (2017JD027556) doi: 10.1002/2017JD027556352
Lawrence, Z. D., Manney, G. L., & Wargan, K. (2018). Reanalysis intercomparisons353
of stratospheric polar processing diagnostics. Atmos. Chem. Phys., 18 , 13547–354
13579. doi: 10.5194/acp-18-13547-2018355
Lawrence, Z. D., Perlwitz, J., Butler, A. H., Manney, G. L., Newman, P. A.,356
Lee, S. H., & Nash, E. R. (2020). The remarkably strong Arctic strato-357
–8–